Ramping Up GMP-Compliant CAR-NK Manufacturing At Scale With iPSCs
By Jack (Jie) Huang, MD, Ph.D., chief scientist and founder, CSTEAM Biotechnology
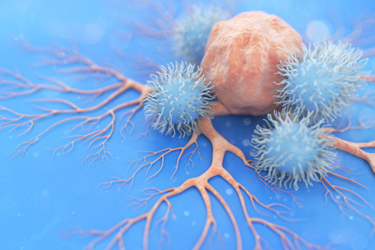
The advancement of cell-based immunotherapies, particularly NK cell therapies, has generated considerable excitement in oncology. However, a major bottleneck to their widespread adoption lies in the complexities of manufacturing these living drugs at clinical and commercial scales.1 Traditional NK cell therapies are typically derived from peripheral blood or umbilical cord blood, which necessitate repeated donor collections and extensive ex vivo expansion protocols. This process introduces significant donor-to-donor variability, limits cell yield, and creates batch inconsistencies that complicate regulatory approval and limit scalability. Moreover, the variability in donor health and immune cell profiles affects both the quality and function of NK cells, creating challenges in achieving consistent therapeutic efficacy.2
This is the second part of a two-part discussion on the advantages and challenges of using and producing iPSC-derived CAR-NK therapies. Click to read part 1.
In contrast, induced pluripotent stem cell (iPSC) technology provides a revolutionary platform for overcoming these limitations. iPSCs, generated from somatic cells through reprogramming, offer the capacity for unlimited self-renewal and the potential to differentiate into virtually any cell type — including NK cells.3 By selecting a single, well-characterized iPSC clone and creating a master cell bank under current Good Manufacturing Practice (cGMP) conditions, researchers can establish a standardized source of NK cells that eliminates the inconsistencies of donor-derived materials.4 From this clone, iPSCs can be reliably expanded and differentiated into NK cells under defined, serum-free, feeder-free conditions, resulting in a homogeneous population of highly functional NK cells with consistent phenotypes and cytotoxic profiles.5
To translate this potential into real-world clinical impact, scalable bioprocessing solutions are critical. Recent innovations have led to the development of bioreactor systems — such as stirred-tank and perfusion bioreactors — that support the controlled expansion and differentiation of iPSCs into NK cells.6 These systems enable precise regulation of essential parameters including pH, dissolved oxygen, temperature, and nutrient levels, which are key to preserving cell integrity, ensuring reproducibility, and maximizing yield.7 The closed, automated nature of bioreactor platforms also enhances GMP compliance by reducing contamination risks and enabling tight quality control throughout the process.
The ability to produce billions of NK cells in a single production run represents a transformative shift in the economics and logistics of cell therapy. This industrialized manufacturing process reduces the cost per dose, facilitates rapid batch release, and makes therapies more accessible to a broader patient population.8 Additionally, iPSC-derived NK cells can be genetically engineered to express chimeric antigen receptors (CARs), transforming them into CAR-NK cells with enhanced specificity and tumor-killing capabilities. These cells can be cryopreserved and stored long-term without loss of viability or function, simplifying inventory management and supporting centralized manufacturing models with global distribution potential.9
Altogether, the integration of iPSC technology with scalable, GMP-compliant manufacturing platforms positions CAR-NK therapies as a next-generation solution in oncology. By overcoming the hurdles of donor dependency, batch variability, and high production costs, iPSC-derived CAR-NK cell therapies are poised to meet the demands of both clinical scalability and commercial feasibility — ushering in a new era of standardized, off-the-shelf cancer immunotherapy.
Figure 1. Scalable IPSC-derived CAR-NK cell therapy
This figure illustrates the workflow for generating therapeutic NK cells from induced pluripotent stem cells (iPSCs). First, a genetically modified iPSC master line encoding a tumor-targeting chimeric antigen receptor (CAR) is created. These engineered iPSCs are expanded in a bioreactor and differentiated into CAR-expressing NK cells under GMP-compliant conditions. The resulting CAR-NK cells are cryopreserved and stored as an “off-the-shelf” therapeutic product. Following patient administration, these cells exhibit targeted cytotoxicity against cancer cells. Scalable, standardized processes enable broad clinical adoption and global distribution of cell-based immunotherapies.
Compliance With GMP And Regulatory Standards
Ensuring GMP compliance in scalable iPSC-derived CAR-NK cell manufacturing
As these therapies move toward clinical application, adherence to good manufacturing practice (GMP) standards is critical. GMP-compliant manufacturing ensures that cellular products are consistently produced and controlled to quality standards suitable for human therapeutic use. To achieve this goal, iPSC-derived CAR-NK cell manufacturing platforms are increasingly adopting automated closed-system workflows to reduce the risk of contamination and human error.10 Process automation not only improves safety and consistency but also facilitates real-time process monitoring and traceability, which are key regulatory requirements.
Comprehensive quality control measures are integrated into every stage of manufacturing, from iPSC storage to genetic modification and differentiation and final product formulation. These measures include sterility testing, genetic stability assays, immunophenotyping, cytotoxicity testing, and assessment of transgene expression levels.11 Regulatory agencies such as the FDA and EMA also require detailed documentation on cell source, genome editing methods, vector safety, and product characteristics before granting research or commercial approval. In addition, as the global demand for iPSC-derived therapies continues to grow, international harmonization of GMP standards is critical to facilitate global clinical trials and product distribution. Companies are increasingly complying with ICH guidelines and seeking certification from multiple regional regulatory agencies.12 By combining advanced manufacturing technologies with a strong regulatory framework, the field is moving toward widespread clinical application and commercialization of iPSC-derived CAR-NK therapies.
Enhanced Therapeutic Consistency and Applicability
Manufacturing iPSC-derived NK cells under good manufacturing practice (GMP) conditions enables superior quality control, reproducibility, and safety monitoring compared to donor-derived alternatives.13 By using well-defined feeder-free culture systems, manufacturers can control critical differentiation steps and eliminate the risk of contamination or unpredictable immune responses. This is particularly important for allogeneic therapies, where immune compatibility and the risk of graft-versus-host disease (GvHD) must be strictly managed. Furthermore, the ability to cryopreserve iPSC-derived NK cells without significant loss of viability or functionality supports flexible clinical logistics. The cells can be produced in large quantities, tested for safety and efficacy, and stored for future use, making them ideal for widespread clinical application. This approach not only supports patient access to treatment but also reduces the costs associated with the production of personalized cell therapies.14
The iPSC-derived NK cells offer a powerful solution that can overcome the key limitations of donor-derived NK therapies. Their scalability, consistency, and genetic flexibility provide a powerful platform for the development of next-generation immunotherapies with improved safety, efficacy, and accessibility in both hematologic and solid tumors.15 As the field continues to mature, iPSC-derived NK cells are expected to become central to the industrialization of cell-based cancer therapies.
Clinical Progress And Preclinical Insights
Preclinical evaluation of iPSC-derived CAR-NK cells
Preclinical studies have laid a solid foundation for the therapeutic application of iPSC-derived CAR-NK cells, demonstrating potent antitumor activity in both hematological malignancies and solid tumors. In vitro studies have shown that iPSC-derived NK cells engineered with a chimeric antigen receptor targeting CD19 exhibit potent cytotoxic activity against B-cell leukemia and lymphoma cell lines.16 These cells exhibited efficient degranulation, high levels of IFN-γ production, and rapid tumor cell lysis. These findings were further confirmed in in vivo xenograft models, in which iPSC-derived CD19 CAR-NK cells significantly reduced tumor burden and prolonged survival without inducing GvHD or severe toxicity.17 In addition to targeting CD19, preclinical studies have also explored CAR-NK constructs targeting solid tumor-associated antigens such as HER2, EGFR, and mesothelin. iPSC-derived CAR-NK cells targeting HER2 have demonstrated successful trafficking to tumor sites, infiltration into the tumor microenvironment, and cytolytic activity against HER2-positive breast and gastric cancer xenografts.18 These findings support the feasibility of iPSC-derived CAR-NK therapies for a variety of cancers and highlight the importance of optimizing trafficking, persistence, and tumor penetration, particularly for solid tumors.
Ongoing clinical trials and early discovery
Several early clinical trials are underway to evaluate the safety and efficacy of iPSC-derived CAR-NK cell therapies in humans. A landmark trial sponsored by Fate Therapeutics (FT596) utilized iPSC-derived CD19-targeted CAR-NK cells that co-expressed IL-15 and CD16 Fc receptors and showed promising early results in patients with relapsed or refractory B-cell lymphoma.19 Preliminary data suggest that a subset of patients had objective tumor responses with minimal adverse events and no evidence of cytokine release syndrome (CRS) or neurotoxicity — complications commonly associated with CAR-T cell therapy.20 Other trials are investigating the use of iPSC-derived CAR-NK cells in multiple myeloma, acute lymphoblastic leukemia, and HER2-positive solid tumors. These studies emphasize not only tumor eradication but also safety, dosing schedule, and persistence of NK cells in the circulation. Importantly, the off-the-shelf nature of iPSC-derived products allows for rapid initiation of therapy and avoids the logistical limitations of autologous cell therapy, making them ideal for urgent clinical situations.21
Advantages Observed In Clinical Translation
One of the most notable advantages observed in both preclinical and clinical settings is the reduced risk of severe immune-related toxicity. Unlike CAR T cells, which often induce CRS and require hospitalization, iPSC-derived CAR-NK cells produce a more controlled immune response.22
The absence of GvHD, even in a fully allogeneic setting, further supports their suitability for universal donor therapy and opens the door to a wider range of patient eligibility. Furthermore, incorporation of IL-15 into CAR-NK constructs has been shown to improve cell persistence in vivo, a key determinant of therapeutic durability.23
Clinical experience also highlights the feasibility of manufacturing, storing, and delivering iPSC-derived CAR-NK cells as cryopreserved ready-to-use products. This logistical advantage reduces treatment delays and improves accessibility for patients in remote or resource-limited settings. Furthermore, standardized manufacturing ensures consistent cell product quality, which is critical for regulatory approval and large-scale commercialization.24
Both preclinical models and early clinical trials support the therapeutic promise of iPSC-derived CAR-NK cells. With demonstrated efficacy, reduced toxicity, and scalable manufacturing, these therapies are moving toward broader clinical application. Continued studies in larger, multicenter trials will determine their long-term safety, optimal dosing strategies, and comparative efficacy with existing treatments. As the field develops, iPSC-derived CAR-NK therapy is expected to become an important component of the next generation of cancer immunotherapy.
Future Outlook: Allogeneic And Universal Therapies
Designing universal iPSC-derived CAR-NK cells
To achieve broad and equitable access to CAR-NK therapies, the development of universal iPSC-derived CAR-NK cell products is a major focus in the field. Unlike autologous therapies that require personalized cell preparation, universal off-the-shelf products can be administered to any patient without the need for HLA matching, significantly reducing production time and cost.
One of the major challenges in achieving this goal is host immune rejection of the graft. To address this issue, gene editing technologies (e.g., CRISPR/Cas9) have been used to disrupt the expression of immunogenic markers, including β2-microglobulin (to eliminate HLA class I) and CIITA (to suppress HLA class II).25
These modifications minimize recognition by recipient T cells and increase the likelihood that the cells will persist in the body for a long time. In addition, researchers are inserting immune evasion transgenes, such as HLA-E or CD47, to further protect CAR-NK cells from host immune responses and clearance by phagocytes.26 Such enhancements make iPSC-derived CAR-NK cells more amenable to allogeneic use and reduce the risk of adverse immune responses, paving the way for truly universal cell therapies. Universal NK cells also allow for the establishment of large cryopreserved cell banks from a single edited iPSC clone, supporting global therapeutic distribution and clinical trial scalability.27
Combination strategies and therapeutic integration
To improve efficacy in refractory cancers, iPSC-derived CAR-NK therapies are increasingly being combined with other modalities. For example, combining CAR-NK cells with immune checkpoint inhibitors such as anti-PD-1 or anti-TIGIT can enhance antitumor function by counteracting inhibitory signals in the tumor microenvironment.28 Oncolytic viruses designed to stimulate local immune responses and increase antigen presentation are also being tested with CAR-NK therapies, particularly in solid tumor models.29 In addition, antibody-based therapies, including bispecific antibodies and monoclonal antibodies, can synergize with NK cells through Fc receptor binding, thereby achieving antibody-dependent cellular cytotoxicity (ADCC).30 This integrated approach can not only enhance NK cell function but also expand the range of tumor targets, providing the potential for personalized combination regimens. As these strategies continue to develop, iPSC-derived CAR-NK cells are expected to become a versatile foundation platform for next-generation tumor therapies.
Conclusion
iPSC-derived CAR-NK cell therapy is emerging as a transformative innovation in cancer immunotherapy, offering a scalable off-the-shelf solution that overcomes the limitations of donor-dependent and autologous-derived therapies. The platform leverages the pluripotency and unlimited self-renewal capacity of iPSCs to enable large-scale production of homogenous NK cells with consistent function and phenotype. Through precise genetic engineering, these NK cells can be equipped with tumor-targeting CARs, enhanced survival signals (e.g., membrane-bound IL-15), and immune escape mechanisms that enable them to persist and function in the immunosuppressive tumor microenvironment.
Unlike traditional T cell therapies that are patient-specific and time-consuming to produce, iPSC-derived CAR-NK therapies are produced using a single, well-characterized master cell bank, ensuring product consistency and reducing variability between different treatment batches. This off-the-shelf solution improves the accessibility of CAR-NK cells, shortens treatment time, and significantly reduces production costs. In addition, the safety of CAR-NK cells, marked by a lower incidence of cytokine release syndrome and neurotoxicity, makes them an ideal alternative or supplement to CAR-T therapy, especially for solid tumors and recurrent hematological malignancies.
Advances in bioreactor design, closed system automation, and compliance with global GMP standards are accelerating the transition of CAR-NK cells from the research stage to clinical and commercial production. Preclinical studies and early clinical trials have shown good safety and efficacy. With the continued innovation of synthetic biology, genome editing, and combination therapy, iPSC-derived CAR-NK cells are expected to become the cornerstone of the next generation of cancer treatment worldwide and provide scalable, effective, and accessible immunotherapy for various tumor indications.
This is the second part of a two-part discussion on the advantages and challenges of using and producing iPSC-derived CAR-NK therapies. Read part 1 here.
References:
- Yinghong Shi et al., Natural killer cell-based cancer immunotherapy: from basics to clinical trials. Experimental Hematology & Oncology 2024 (https://doi.org/10.1186/s40164-024-00561-z)
- Melissa Berrien-Elliott et al., Allogeneic natural killer cell therapy. Blood 2023 (https://doi.org/10.1182/blood.2022016200)
- Jonas Cerneckis et al., Induced pluripotent stem cells (iPSCs): molecular mechanisms of induction and applications. Signal Transduction and Targeted Therapy 2024 (https://doi.org/10.1038/s41392-024-01809-0)
- Mahendra Rao et al., Illustrating the potency of current Good Manufacturing Practice–compliant induced pluripotent stem cell lines as a source of multiple cell lineages using standardized protocols. Cytotherapy 2018 (https://doi.org/10.1016/j.jcyt.2018.03.037)
- Wenhua Qiao et al., Advances in induced pluripotent stem cell-derived natural killer cell therapy. Cells 2024 (doi: 10.3390/cells13231976)
- Lila Rutte et al., Advancing Translation of Clinical Research Into Practice and Population Health Impact Through Implementation Science. Mayo Clinic Proceedings 2024 (DOI: 10.1016/j.mayocp.2023.02.005)
- Chee Keong Kwok et al., Scalable expansion of iPSC and their derivatives across multiple lineages. Reproductive Toxicology 2022 (https://doi.org/10.1016/j.reprotox.2022.05.007)
- Fang Fang et al., Advances in NK cell production. Cellular & Molecular Immunology 2022 (https://doi.org/10.1038/s41423-021-00808-3)
- Sagnik Mitra and Ganti Murthy, Bioreactor control systems in the biopharmaceutical industry: a critical perspective. Systems Microbiology and Biomanufacturing 2021 (doi: 10.1007/s43393-021-00048-6)
- Clara Sanz-Nogues and Timothy O'Brien, Current good manufacturing practice considerations for mesenchymal stromal cells as therapeutic agents. Biomaterials and Biosystems 2021 (https://doi.org/10.1016/j.bbiosy.2021.100018)
- Huu Du Nguyen et al., Quality control for smart manufacturing in industry 5.0. Artificial Intelligence for Smart Manufacturing 2023 (https://link.springer.com/chapter/10.1007/978-3-031-30510-8_3)
- Gerd Bode, Regulatory guidance: ICH, EMA, FDA. drug discovery and evaluation: methods in clinical pharmacology 2018 (https://link.springer.com/referenceworkentry/10.1007/978-3-319-56637-5_58-1)
- P K J D de Jonge et al., Good manufacturing practice production of CD34+ progenitor-derived NK cells for adoptive immunotherapy in acute myeloid leukemia. Cancer Immunology and Immunotherapy 2023 (doi: 10.1007/s00262-023-03492-6)
- Roisin O'Flaherty et al., Mammalian cell culture for production of recombinant proteins: A review of the critical steps in their biomanufacturing. Biotechnology Advances 2020 (https://doi.org/10.1016/j.biotechadv.2020.107552)
- Benjamin Goldenson et al., iPSC-derived natural killer cell therapies - expansion and targeting. Frontiers in Immunology 2022 (doi: 10.3389/fimmu.2022.841107)
- Xiao Wang et al., Chimeric antigen receptor-engineered NK cells: new weapons of cancer immunotherapy with great potential. Experimental Hematology & Oncology 2022 (https://doi.org/10.1186/s40164-022-00341-7)
- Margery Gang et al., CAR-modified memory-like NK cells exhibit potent responses to NK-resistant lymphomas. Blood 2020 (DOI:10.1182/blood.2020006619)
- Wenxiu Wang et al., CAR-NK for tumor immunotherapy: Clinical transformation and future prospects. Cancer Letters 2020 (https://doi.org/10.1016/j.canlet.2019.11.033)
- Veronika Bachanova et al., Safety and efficacy of FT596, a first-in-class, multi-antigen targeted, off-the-shelf, iPSC-derived CD19 CAR NK cell therapy in relapsed/refractory B-cell lymphoma. Blood 2021 (https://doi.org/10.1182/blood-2021-151185)
- Yize Mao et al., The landscape of objective response rate of anti-PD-1/L1 monotherapy across 31 types of cancer: a system review and novel biomarker investigating. Cancer Immunology and Immunotherapy 2023 (doi: 10.1007/s00262-023-03441-3)
- Yan Zhong and Jingfeng Liu, Emerging roles of CAR-NK cell therapies in tumor immunotherapy: current status and future directions. Cell Death Discovery 2024 (https://doi.org/10.1038/s41420-024-02077-1)
- Yingying Shi et al., Progress and prospects of mRNA-based drugs in pre-clinical and clinical applications. Signal Transduction and Targeted Therapy 2024 (https://doi.org/10.1038/s41392-024-02002-z)
- Katie Maurer and Robert Soiffer, The delicate balance of graft versus leukemia and graft versus host disease after allogeneic hematopoietic stem cell transplantation. Expert Review of Hematology 2024 (doi: 10.1080/17474086.2023.2273847)
- Ya-Ju Chang et al., High-density cryopreservation of off-the-shelf CAR-NK cells facilitates on-demand treatment access. Blood 2022 (https://doi.org/10.1182/blood-2022-162712)
- Torsten Meissner et al., Immune editing: overcoming immune barriers in stem cell transplantation. Current Stem Cell Repports 2022 (doi: 10.1007/s40778-022-00221-0)
- Akitsu Hotta et al., Genetically engineered hypoimmunogenic cell therapy. Nature Reviews Bioengineering 2024 (https://doi.org/10.1038/s44222-024-00219-9)
- Xiaotong Lin et al., IPSC-derived CAR-NK cells for cancer immunotherapy. Biomedicine Pharmacotherapy 2023 (doi: 10.1016/j.biopha.2023.115123)
- Minggang Fang et al., Unlocking the potential of iPSC-derived immune cells: engineering iNK and iT cells for cutting-edge immunotherapy. Frontiers in Immunology 2024 (doi: 10.3389/fimmu.2024.1457629)
- Jonathan Santos Apolonio et al., Oncolytic virus therapy in cancer: A current review. World Journal of Virology 2021 (doi: 10.5501/wjv.v10.i5.229)
- Ashu Shah et al., The Current Landscape of Antibody-based Therapies in Solid Malignancies. Theranostics 2021 (doi: 10.7150/thno.52614)
About The Author:
Jack (Jie) Huang, MD, Ph.D., is the chief scientist, founder, and CEO at CSTEAM Biotechnology in Ohio. He is recognized as a medical science writer, an inventor, and a STEM educator. Huang completed his MD and Ph.D. at Shiga University of Medical Science (SUMS) in Japan in 2000. He then pursued postdoctoral training in immunology at the University of Tokyo, followed by a role as research assistant professor at Tohoku University. In the U.S., he served as a postdoctoral fellow at Oregon Health & Science University and as an oncologist and senior research scientist at Ohio State University Nationwide Children’s Hospital, focusing on oncology research until 2018, when he launched his company specializing in biological models and biochips. Additionally, Huang was vice president of the American Botanical Drug Association, director of the Stem Cell Engineering and Technology Research Center at the Industry Technology Research Institute of Chongqing University, and chief scientist of the International Institute of In Vitro Diagnostics at Chongqing Medical University. His research interests, which span genetic engineering biological models, gene-edited stem cells, immune cell drugs, and biochip technology, have led to numerous patents.