How CAR-NKs Offer Powerful Therapeutic Flexibility
By Jack (Jie) Huang MD, Ph.D., chief scientist, founder, and CEO, CSTEAM Biotechnology
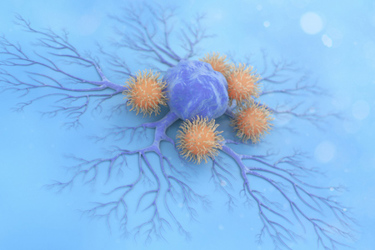
Chimeric antigen receptor (CAR) technology has revolutionized the landscape of cancer immunotherapy, particularly with the success of CAR T cell therapy in treating hematologic malignancies such as B-cell acute lymphoblastic leukemia and diffuse large B-cell lymphoma. Despite its clinical efficacy, CAR-T therapy faces several limitations, including high manufacturing costs, severe side effects such as cytokine release syndrome (CRS) and neurotoxicity, limited persistence in some patients, and the risk of graft-versus-host disease (GvHD) in the allogeneic setting.1 These challenges have led to growing interest in natural killer (NK) cells as a safer and more scalable alternative to CAR therapy.
NK cells are innate immune effector cells that can eliminate tumor cells without prior sensitization or reliance on antigen presentation via major histocompatibility complex (MHC) molecules.2 They are naturally cytotoxic and have a low risk of GvHD and cytokine storm, making them attractive candidates for allogeneic cell therapy.3 However, primary NK cells derived from peripheral blood or umbilical cord blood exhibit limitations in terms of expansion capacity, functional heterogeneity, and short in vivo duration, which restricts their clinical and commercial viability.4
This is the first part of a two-part discussion on the advantages and challenges of using and producing iPSC-derived CAR-NK therapies. Click to read part 2.
To overcome these obstacles, induced pluripotent stem cell (iPSC)-derived NK cells have emerged as a promising platform for scalable production of CAR-engineered NK cells. iPSCs can self-renew indefinitely and differentiate into nearly any type of somatic cell, including NK cells, under specific culture conditions.5 This unique property enables the generation of a renewable, standardized, and genetically engineered source of NK cells that can be expanded to large quantities. Unlike donor-dependent primary NK cells, iPSC-derived NK cells have batch-to-batch consistency and homogeneity, which is critical for clinical reproducibility and industrial manufacturing.6
Genetic engineering techniques can stably integrate CAR constructs into iPSC lines, allowing the development of CAR-NK cells that can target tumor-specific antigens such as CD19, HER2, and EGFR.7 Furthermore, co-expression of cytokines such as IL-15 or checkpoint blockade molecules has been shown to enhance in vivo persistence and resistance to tumor-induced immunosuppression.8 Importantly, iPSC-derived CAR-NK cells have demonstrated potent cytotoxic responses in preclinical models of both hematological and solid tumors, with minimal off-target effects and a low incidence of serious immune-related adverse events.9
The scalability of iPSC-derived CAR-NK cells is a significant advantage over traditional autologous approaches. From a single engineered iPSC clone, billions of CAR-NK cells can be produced in a GMP compliant manner using bioreactor-based culture systems.10 This enables the creation of off-the-shelf cell therapy products that can be stored, shipped, and administered without customization to the patient. Early clinical trials are already underway to evaluate the safety and efficacy of iPSC-derived CAR-NK cells in humans, with preliminary data demonstrating promising antitumor activity and excellent tolerability.11
With the advancement of research and technology, scalable iPSC-derived CAR-NK cell therapy has the potential to become the cornerstone of the next generation of cancer immunotherapy. With its ability to standardize production and reduce toxicity and its broad applicability, it addresses many limitations of current CAR-T therapies and paves the way for accessible and effective cell therapies.
Figure 1. Key strategies enhancing the therapeutic potential of CAR-NK cell therapy
This figure highlights four major advantages of CAR-NK cell therapy in cancer treatment. First, CAR-NK cells can effectively target a variety of tumor antigens, including those from hematological malignancies and solid tumors. Second, genetic modification enhances its therapeutic potential through cytokine support, immune checkpoint disruption, and immune escape. Third, CAR-NK cells can be used in combination with other therapies, such as anti-cancer antibodies, oncolytic viruses, and checkpoint inhibitors. Finally, its allogeneic adaptability enables it to be applied to a wide range of patient populations through a single engineered cell source.
Overcoming The Limitations Of Primary NK Cells
Challenges associated with donor-derived NK cells
NK cells are an important component of the innate immune system and are responsible for the rapid elimination of virally infected and malignant cells through mechanisms such as direct cytotoxicity, cytokine production, and antibody-dependent cellular cytotoxicity (ADCC).12 They mediate antitumor activity without prior antigen sensitization, making them attractive candidates for cancer immunotherapy. However, the clinical application of primary NK cells (usually derived from peripheral blood, umbilical cord blood, or haploidentical donors) faces significant challenges. A major limitation is their short in vivo persistence. After infusion, donor-derived NK cells often fail to survive long enough to achieve a sustained therapeutic effect, especially in the immunosuppressive tumor microenvironment.13 In addition, they have limited proliferation potential in vitro, and expansion protocols often generate heterogeneous populations with different cytotoxic profiles and inconsistent phenotypes.14 The diversity of donors further complicates their use. The quality and function of NK cells depends largely on the source and characteristics of the donor, such as age, health status, and previous immune exposure. These factors can lead to inconsistent therapeutic manufacturing and patient outcomes.15 Furthermore, the repeated leukapheresis procedures required for cell collection can pose logistical and ethical issues, particularly when scaling up treatments for large patient populations. For clinical-grade therapies, the diversity of donor-derived NK cells presents barriers to standardization, reproducibility, and regulatory approval.
Advantages of iPSC-derived NK cells
To address these limitations, the generation of NK cells from iPSCs has emerged as a promising alternative. iPSCs can self-renew indefinitely and differentiate into any somatic cell lineage, including NK cells, under specific culture conditions.16 This provides a virtually unlimited source of NK cells that can be generated from a single master iPSC line, ensuring consistency in phenotypic and functional properties between production batches. Such standardization is critical to ensure reliable clinical performance and scalable manufacturing. Unlike donor-derived NK cells, iPSC-derived NK cells can be expanded into large quantities in bioreactor systems and stored for ready use. Importantly, iPSCs can be genetically engineered at the pluripotent stage to achieve stable and controlled integration of enhanced functionality, such as cytokine support (e.g., IL-15 expression), immune checkpoint resistance (e.g., PD-1 knockout), and tumor-targeting receptors (e.g., CARs).17 This allows for the creation of highly optimized NK cell products that target specific malignancies while avoiding many of the pitfalls associated with in vitro engineering of mature NK cells.
CAR-NK Cell Tumor Targeting Engineering
Principles of CAR engineering in NK cells
The CAR is a synthetic fusion protein that endows immune cells with the ability to recognize and kill cancer cells by targeting specific tumor-associated antigens (TAAs). Although CAR technology has been successfully applied to T cells, its application in NK cells, especially those derived from iPSCs, requires tailored modifications based on the unique signaling and cytotoxic mechanisms of NK cells. CARs are typically composed of an antibody-derived extracellular single-chain variable fragment (scFv) that binds to the TAA, a transmembrane domain, and an intracellular signaling domain that activates immune cell cytotoxicity upon antigen engagement.18
In T cells, CARs traditionally contain the CD3ζ chain and a co-stimulatory domain such as CD28 or 4-1BB. However, NK cells utilize different activation pathways. Therefore, NK-specific CAR constructs have been designed with alternative signaling domains (e.g., DAP10, DAP12, and 2B4) that are more physiologically appropriate for NK cell activation and function.19 These modifications help ensure a robust NK cell response upon CAR binding, including degranulation, cytokine production, and target cell killing.
Target antigen selection and tumor specificity
Target selection is an important aspect of CAR engineering, particularly for avoiding off-tumor cytotoxicity. The most widely used antigen in hematological malignancies is CD19, which is expressed in B-cell leukemias and lymphomas. iPSC-derived CAR-NK cells engineered to express anti-CD19 CARs have demonstrated potent tumor clearance in preclinical models with reduced toxicity compared to CAR T cells.20
For solid tumors, CAR constructs targeting HER2, EGFR, and mesothelin have shown promise. However, tumor antigen heterogeneity and low expression in healthy tissues remain significant challenges. Specificity, affinity modulation, and dual-targeting strategies for scFv selection are being explored to improve selectivity and reduce the risk of off-target effects.21 To improve precision, researchers are investigating logic-gated CARs and synthetic Notch receptors, which require co-expression of two antigens prior to activation to limit cytotoxicity to malignant cells. These advanced constructs can be integrated into iPSC lines at an early stage of differentiation, allowing the development of safer tumor-specific CAR-NK therapies.22
Cytokine support improves NK cell function
Conventional NK cell therapies are limited in their survival and proliferation in vivo. This problem is particularly pressing in the solid tumor setting, as NK cells must survive and function in a harsh microenvironment. To overcome this problem, cytokine support systems have been incorporated into CAR-NK cells to promote autonomous survival. IL-15 is one of the most studied cytokines. By engineering iPSC-derived CAR-NK cells to co-express membrane-bound or secreted IL-15, researchers have significantly improved their persistence and cytotoxic activity without the need for exogenous cytokine administration.23 IL-15 not only supports survival but also enhances NK cell metabolic fitness, proliferation, and cytotoxicity. Importantly, IL-15 expression must be carefully regulated, as uncontrolled cytokine release may increase the risk of toxicity. Various strategies, including inducible promoters or transient expression systems, are being developed to fine-tune cytokine levels in vivo and maintain a favorable therapeutic index.24
Enhanced resistance to immunosuppression
Tumors often develop mechanisms to evade immune surveillance by exploiting immune checkpoint pathways or secreting immunosuppressive factors such as TGF-β, adenosine, and IDO. A key pathway involves the interaction of PD-1 on immune cells with PD-L1 on tumor cells, leading to functional failure. To counteract this, CAR-NK cells can be engineered with PD-1 knockout or dominant negative PD-1 receptors, rendering them resistant to tumor-mediated suppression.25 In addition, iPSC-derived CAR-NK cells can be modified to express cytokine receptors or transcription factors that confer TGF-β resistance or promote adaptation to oxidative stress. These modifications enable NK cells to remain active in the suppressive tumor microenvironment. This enhancement is critical for the efficacy of CAR-NK therapy in solid tumors, which present a more complex and suppressive immune landscape than hematological malignancies.26
Integration of gene editing and synthetic biology
The use of genome editing technologies, especially CRISPR/Cas9, has greatly facilitated the precise engineering of iPSC-derived NK cells. Gene editing allows the insertion of CAR constructs into safe harbor sites such as AAVS1 or CCR5, ensuring stable and uniform expression. This avoids issues associated with viral vector integration, such as insertional mutagenesis or variable CAR expression.27 In addition, synthetic biology tools are being used to create “smart” CAR-NK cells that respond dynamically to tumor signals. For example, researchers have developed switchable CARs that can be turned on or off using small molecules, as well as feedback control circuits that can regulate cytokine expression in response to environmental cues.28 These technologies have improved the precision, safety, and adaptability of CAR-NK cells, making them applicable to a wider range of cancers and patient populations.
Conclusion
CAR-NK cell therapy represents a rapidly developing frontier in cancer immunotherapy, with unique advantages over traditional CAR-T therapy. One of the most important breakthroughs is the development of iPSC-derived CAR-NK cells, which addresses the challenges of donor variability, limited expansion capacity, and short in vivo persistence in primary NK cells. iPSC technology enables the production of standardized, off-the-shelf NK cells and enhances their scalability and reproducibility, making them more suitable for a wide range of clinical applications.
The engineering of CAR-NK cells has progressed through multidisciplinary innovations aimed at improving tumor targeting, cytotoxic potency, and resistance to immunosuppressive tumor microenvironments. Novel CAR structures optimized for NK cell signaling, the integration of cytokine support elements such as IL-15, and precision gene editing tools such as CRISPR-Cas9 have collectively improved the functionality and persistence of CAR-NK. These engineered cells not only exhibit potent anti-tumor activity but also reduce the risk of cytokine release syndrome and neurotoxicity commonly seen in CAR-T therapy. In addition, CAR-NK cells have shown good efficacy in the treatment of both hematological malignancies and solid tumors, and they have overcome the problems of tumor heterogeneity and antigen escape through dual-targeting strategies and "armored" NK cell platforms. With the maturity of production technology and the expansion of clinical trials, CAR-NK therapy is expected to become the cornerstone of the next generation of immunotherapy. Its ready potential, safety, and adaptability make it a powerful tool to fight cancer and can flexibly respond to various treatment challenges in precision oncology.
Looking ahead, CAR-NK therapy is expected to provide a scalable, off-the-shelf cancer treatment method with greater safety, precise targeting, and wide applicability, making it an important force in future immuno-oncology. Part 2 of this discussion explores the highly scalable nature of iPSC-derived CAR-NK cells.
References:
- Samane Abbasi et al., Chimeric antigen receptor T (CAR-T) cells: Novel cell therapy for hematological malignancies. Cancer Medicine 2023 (DOI: 10.1002/cam4.5551)
- Arosh Shavinda Perera Molligoda Arachchige, Human NK cells: From development to effector functions. Innate Immunity 2021 (doi: 10.1177/17534259211001512)
- Carlijn Voermans and Mette Hazenberg, Cellular therapies for graft-versus-host disease: a tale of tissue repair and tolerance. Blood 2020 (https://doi.org/10.1182/blood.2019000951)
- Chantal Reina-Ortiz et al., Expanded NK cells from umbilical cord blood and adult peripheral blood combined with daratumumab are effective against tumor cells from multiple myeloma patients. Oncoimmunology 2020 (doi: 10.1080/2162402X.2020.1853314)
- Keerthana Shankar et al., Genome engineering of induced pluripotent stem cells to manufacture natural killer cell therapies. Stem Cell Research & Therapy 2020 (doi: 10.1186/s13287-020-01741-4)
- J Moscarelli et al., The next generation of cellular immunotherapy: CAR-NK cells. Transplant Cell Therapy 2022 (doi: 10.1016/j.jtct.2022.06.025)
- Ying Gong et al., Chimeric antigen receptor natural killer (CAR-NK) cell design and engineering for cancer therapy. Journal of Hematology & Oncology 2021 (https://doi.org/10.1186/s13045-021-01083-5)
- Jian Qiao and Yang-Xin Fu, Cytokines that target immune killer cells against tumors. Cellular & Molecular Immunology 2020 (doi: 10.1038/s41423-020-0481-0)
- Linqin Wang et al., CD70-targeted iPSC-derived CAR-NK cells display potent function against tumors and alloreactive T cells. Cell Reports Medicine 2025 (https://doi.org/10.1016/j.xcrm.2024.101889)
- Son Hai Vu and Xuan-Hung Nguyen, Advancing cancer immune cell therapies via engineered iPSC-based strategies. Interdisciplinary Cancer Research 2024 (https://link.springer.com/chapter/10.1007/16833_2024_293)
- Rui Yang et al., Advances in CAR-NK cell therapy for hematological malignancies. Frontiers in Immunology 2024 (https://doi.org/10.3389/fimmu.2024.1414264)
- Arundhati Mandal and Chandra Viswanathan, Natural killer cells: In health and disease. Hematology/Oncology and Stem Cell Therapy 2015 (https://doi.org/10.1016/j.hemonc.2014.11.006)
- Raynier Devillier et al., Phase I trial of prophylactic donor-derived IL-2-activated NK cell infusion after allogeneic hematopoietic stem cell transplantation from a matched sibling donor. Cancers 2021 (doi: 10.3390/cancers13112673)
- Yavor Bozhilov et al., In vitro human haematopoietic stem cell expansion and differentiation. Cells 2023 (doi: 10.3390/cells12060896)
- Catherine Harbour et al., How donors can collaborate to improve reach, quality, and impact in social and behavior change for health. Globe Health: Science and Practice 2021 (doi: 10.9745/GHSP-D-21-00007)
- Kyle Lupo et al., Differentiation of natural killer cells from induced pluripotent stem cells under defined, serum- and feeder-free conditions. Cytotherapy 2021 (DOI: 10.1016/j.jcyt.2021.05.001)
- Alex Nianias and Maria Themeli, Induced pluripotent stem cell (iPSC)–derived lymphocytes for adoptive cell immunotherapy: recent advances and challenges. Current Hematologic Malignancy Reports 2019 (doi: 10.1007/s11899-019-00528-6)
- Nico Sievers et al., CARs: beyond T cells and T cell-derived signaling domains. International Journal of Molecular Sciences 2020 (doi: 10.3390/ijms21103525)
- Emma McErlean and Helen McCarthy, Non-viral approaches in CAR-NK cell engineering: connecting natural killer cell biology and gene delivery. Journal of Nanobiotechnology 2024 (https://doi.org/10.1186/s12951-024-02746-4)
- Jianshu Wei et al., Target selection for CAR-T therapy. Journal of Hematology & Oncology 2019 (doi: 10.1186/s13045-019-0758-x)
- Amin Daei Sorkhabi et al., The current landscape of CAR T-cell therapy for solid tumors: Mechanisms, research progress, challenges, and counterstrategies. Frontiers in Immunology 2023 (doi: 10.3389/fimmu.2023.1113882)
- Simon Fraessle et al., Activation-inducible CAR expression enables precise control over engineered CAR T cell function. Communications Biology 2023 (https://doi.org/10.1038/s42003-023-04978-w)
- Guangyu Lian et al., Challenges and recent advances in NK cell-targeted immunotherapies in solid tumors. International Journal of Molecular Sciences 2021 (doi: 10.3390/ijms23010164)
- Sogand Vahidi et al., IL-15 as a key regulator in NK cell-mediated immunotherapy for cancer: From bench to bedside. International Immunopharmacology 2024 (https://doi.org/10.1016/j.intimp.2024.112156)
- Shovan Dutta et al., Targets of Immune Escape Mechanisms in Cancer: Basis for Development and Evolution of Cancer Immune Checkpoint Inhibitors. Biology 2023 (doi: 10.3390/biology12020218)
- Xiaotong Lin et al., IPSC-derived CAR-NK cells for cancer immunotherapy. Biomedicine Pharmacotherapy 2023 (doi: 10.1016/j.biopha.2023.115123)
- Alaa Aljabali et al., Principles of CRISPR-Cas9 technology: Advancements in genome editing and emerging trends in drug delivery. Journal of Drug Delivery Science and Technology 2024 (https://doi.org/10.1016/j.jddst.2024.105338)
- Justin Clubb et al., Synthetic biology in the engineering of CAR-T and CAR-NK cell therapies: facts and hopes. Clinical Cancer Research 2023 (doi: 10.1158/1078-0432.CCR-22-1491)
About The Author:
Jack (Jie) Huang, MD, Ph.D., is the chief scientist, founder, and CEO at CSTEAM Biotechnology in Ohio. He is recognized as a medical science writer, an inventor, and a STEM educator. Huang completed his MD and Ph.D. at Shiga University of Medical Science (SUMS) in Japan in 2000. He then pursued postdoctoral training in immunology at the University of Tokyo, followed by a role as research assistant professor at Tohoku University. In the U.S., he served as a postdoctoral fellow at Oregon Health & Science University and as an oncologist and senior research scientist at Ohio State University Nationwide Children’s Hospital, focusing on oncology research until 2018, when he launched his company specializing in biological models and biochips. Additionally, Huang was vice president of the American Botanical Drug Association, director of the Stem Cell Engineering and Technology Research Center at the Industry Technology Research Institute of Chongqing University, and chief scientist of the International Institute of In Vitro Diagnostics at Chongqing Medical University. His research interests, which span genetic engineering biological models, gene-edited stem cells, immune cell drugs, and biochip technology, have led to numerous patents.