4 Key Solid Tumor Therapy Objectives iPSCs Can Help Achieve
By Shubhranshu Gupta, Ph.D., cell therapy expert
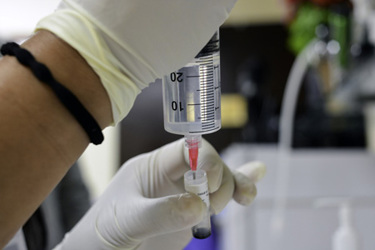
The development of cell therapy products for solid tumors represents one of the most promising yet challenging pursuits in medicine. Solid tumors account for a significant portion of cancer-related deaths, with mortality rates ranging from 50% to 70%, depending on tumor type and stage.¹ Relapse rates for advanced solid tumors often exceed 50%, driven by mechanisms such as tumor dormancy and antigen escape.2,3 These statistics underscore the urgent need for therapies that can improve survival outcomes and reduce recurrence.
While CAR-T therapies have transformed blood cancer care, adapting these approaches to solid tumors remains highly complex. Emerging CAR-NK therapies offer additional promise by leveraging natural killer cells' innate ability to target cancer cells while reducing risks such as cytokine release syndrome and neurotoxicity that have typically been observed for CAR-T therapies derived from peripheral blood mononuclear cells (PBMC).4
Although PBMC-derived cell therapies have shown promise, they face significant limitations that impact their effectiveness in treating solid tumors. These therapies are constrained by several key challenges:
- limited gene editing capacity that restricts their ability to address complex tumor environments,
- high risk of cytokine release syndrome and neurotoxicity due to bystander cytokine secretion,
- substantial manufacturing challenges including high costs often exceeding $500,000 per dose,5,6
- and significant batch-to-batch variability.
These high costs make these treatments unaffordable for most patients and inaccessible to rural healthcare organizations that often lack infrastructure and expertise.7,8 Additionally, PBMC-derived therapies struggle with donor-dependent inconsistencies and limited scalability due to their autologous nature.
Induced pluripotent stem cells (iPSCs) have emerged as a groundbreaking platform that directly addresses these limitations. By virtue of their unique biological properties, iPSCs enable extensive multi-gene editing without compromising genetic stability, support scalable off-the-shelf manufacturing with reduced variability, and demonstrate lower risks of bystander cytokine secretion and immune-related adverse events. Their unlimited expansion potential and ability to differentiate into various immune cells make them an ideal source for next-generation cell therapies.
The advantages of iPSC-derived therapies extend beyond overcoming PBMC limitations. Their amenability to genetic engineering allows for the creation of hypo-immunogenic cells that can evade immune detection, while their consistent quality and scalable manufacturing processes address the practical challenges of bringing cell therapies to market. This transformative platform represents a paradigm shift in how we approach solid tumor treatment, offering solutions to both the biological and manufacturing challenges that have historically limited the effectiveness of cell therapies.
The following sections examine how iPSC-derived platforms offer solutions to these long-standing challenges through enhanced genetic engineering capabilities, improved scalability, reduced manufacturing variability, and significantly lower cost per dose.
So Far, Cell Therapy Developers Have Struggled To Infiltrate Solid Tumors
The success of CAR-T and CAR-NK therapies in treating blood cancers has marked a watershed moment in cancer immunotherapy.9 While these therapies have demonstrated remarkable efficacy in hematologic malignancies, their application to solid tumors has revealed significant challenges that demand innovative solutions. Solid tumors present a complex array of obstacles, from their physical and chemical barriers to their immunosuppressive microenvironment, which collectively inhibit immune cell infiltration and activity.10 The heterogeneous nature of solid tumors further complicates treatment by enabling antigen escape mechanisms, leading to therapy resistance and tumor recurrence.10
Current PBMC-derived cell therapies, while promising, face substantial limitations in addressing these challenges. CAR-NK therapies emerged as a potential alternative, offering advantages such as reduced toxicity and the possibility of off-the-shelf availability.12 The manufacturing complexity of these therapies, involving intricate processes like gene transfer and cell expansion, creates additional barriers to scalability and consistency. Moreover, navigating the evolving regulatory frameworks across different jurisdictions adds another layer of complexity to bringing these therapies to market.13
These multifaceted challenges underscore the need for transformative approaches that can address both the biological and manufacturing limitations of current cell therapies. The following section examines how innovative platforms, particularly iPSC-derived solutions help to overcome these long-standing obstacles.
Transforming Solid Tumor Treatment: From Challenges To iPSC-Powered Solutions
The development of cell therapies for solid tumors faces challenges spanning scientific, manufacturing, logistical, and regulatory domains. From overcoming the immunosuppressive tumor microenvironment to navigating the complex manufacturing and regulatory landscape, a concerted effort across disciplines is required to realize these therapies’ potential. For cell therapy to be successful, researchers must achieve four objectives:
- Neutralize the immunosuppressive tumor microenvironment.
- Mitigate antigen escape and enhance CAR-NK and CAR-T persistence through genetic modifications.
- Improve manufacturing scalability with platforms such as iPSCs.
- Overcoming regulatory hurdles across global markets.
1. Tackling Tumor Microenvironment Suppression
The tumor microenvironment (TME) remains a central obstacle, with its immunosuppressive, physical, and metabolic barriers limiting the efficacy of immune cells. Genetic modifications, such as engineering CAR‑T or CAR‑NK cells to express checkpoint inhibition molecules like PD-1 knockouts, have shown efficacy in preclinical models14, and combining cell therapies with immune checkpoint inhibitors (e.g., anti‑PD‑1 or anti‑CTLA‑4 antibodies) can further neutralize tumor suppression.15
In addition, M2 macrophages — driven by tumor-derived factors including hypoxia, lactic acid, IL‑4, IL‑10, TGF‑β, and IL‑13 — and their associated receptor tyrosine kinases (Axl, MerTK) play a pivotal role in sustaining the TME.16-18 Metabolic engineering strategies, such as modifying CAR‑T cells to overexpress glucose transporters like GLUT1, inhibiting lactate dehydrogenase (LDH), using buffering agents, or expressing enzymes like argininosuccinate synthase (ASS), enhance cell survival and cytotoxicity in nutrient-depleted, acidic TME conditions.19-23
All the above-mentioned modifications often require sophisticated multiplexing gene editing using tools to incorporate multiple complex edits into CAR-T or CAR-NK cells without significantly compromising their viability or functionality during expansion. The current state-of-art cell therapy strategies including the examples mentioned above predominantly involve PBMC-derived T or NK cells, which are limited in their capacity for multi-gene editing (up to four edits achieved to date).24
In contrast, iPSCs offer significant advantages: they permit multi‑gene editing without markedly compromising genetic stability, viability, or differentiation. Products like FT825 and FT522 by Fate Therapeutics have demonstrated the feasibility of engineering iPSC-derived T and NK cells with more than five edits.25 Such innovations could shift the paradigm from PBMC‑derived cell therapies to iPSC‑derived platforms, which may better overcome an immunosuppressive TME.
2. Overcoming Tumor Heterogeneity And Antigen Escape
Tumor heterogeneity and antigen escape further complicate treatment. Multi‑antigen targeting strategies — for example, dual CAR‑T cells designed to target both HER2 and IL13Rα2 in glioblastoma — aim to address heterogeneity but risk off‑target toxicity against normal tissues that express low levels of these antigens.26-28
Additionally, persistent antigen stimulation from dual targeting can lead to T cell exhaustion, as evidenced by the upregulation of exhaustion markers like PD-1 and TIM-329; which can potentially limit the long-term efficacy of this approach. Bispecific T‑cell engagers (BiTEs), such as blinatumomab targeting CD19, have shown success in hematological cancers and are under investigation in solid tumors30-32; however, their efficacy is often hindered by limited tumor penetration, short half‑lives, rapid antigen escape, and subsequent T cell exhaustion.33-35
Combination therapies — for example, CAR‑T cells paired with immune checkpoint inhibitors or engineered to secrete BiTEs — can bolster T‑cell functionality,36,37 but may also increase immune‑related adverse events and drive chronic T-cell exhaustion potentially leading to tumor relapse38; necessitating safety switches to manage these risks.
In the ever-progressing ways to overcome tumor antigen-escape via use of bispecific CAR, BiTEs or combination strategies discussed above, the challenge in the field has been to overcome irAEs resulting from cytokine release syndrome (CRS) and Immune effector cell-associated neurotoxicity syndrome (ICANS) often resulting from bystander activation of the host macrophage population via GM-CSF and IFN-γ, whose secretion by PBMC-derived CAR-T cells continues despite disappearance of the cognate tumor antigen.39-44 Such risk of continued bystander cytokine secretion in absence of cognate antigen is significantly lower in iPSC-derived CAR T cells.41
iPSC-derived cells can be engineered to express multiple CARs simultaneously while maintaining genetic stability, providing a more robust approach to addressing tumor heterogeneity. These cells also demonstrate enhanced tumor killing compared to traditional CAR-T cells, partly due to their ability to be precisely engineered for optimal functionality.45 Regardless of the source that CAR-T or CAR-NK cells are derived from, leveraging single-cell sequencing technologies to profile tumor antigens at an unprecedented depth can address the issue of antigen escape more effectively. These insights may enable researchers to identify evolving tumor antigens during the course of cell therapy and adjust therapeutic targets dynamically. By embedding antigen epitope mapping into clinical workflows, cell therapy designs could be refined in real time, ensuring sustained efficacy across heterogeneous tumor populations.
3. Optimizing Manufacturing Scalability, Lowering Cost And Enhancing Accessibility
Scalability, cost reduction, and treatment accessibility are additional challenges. Autologous therapies are labor‑intensive and costly.46 Automation platforms, closed-loop systems for cell isolation, transduction, and expansion — as highlighted by the International Society for Cell Therapy — and “process‑in‑a‑box” solutions can streamline manufacturing.47,48
Stirred-tank bioreactors with dynamic controls, digital twin simulations, and innovative reimbursement models (e.g., outcomes‑based payment) are pivotal for large-scale production and adoption, although these approaches introduce financial risks due to patient heterogeneity. Furthermore, outcomes-based reimbursement models may align payment with clinical value, but they risk manufacturers not receiving full compensation if patients do not respond to treatment or if milestone agreements prove difficult to establish. Logistics further complicate widespread access, requiring robust cryogenic storage and specialized transport to maintain cell viability and ensure proper chain‑of‑identity and chain‑of‑custody, particularly in rural settings.
iPSC‑derived CAR‑T and CAR‑NK therapies offer a transformative alternative. Utilizing a well‑characterized, off‑the‑shelf iPSC master cell bank eliminates the need for patient‑ or donor‑specific collection, reduces variability, and simplifies regulatory compliance. This has encouraged several companies to pioneer this approach on a clinical scale at significantly reduced cost while maintaining functionality and viability.
For example, FT596, an iPSC-derived CAR-NK product, cost $2,500 per dose in its phase I trial.49 Lower production costs would make treatments more accessible globally and reduce reliance on expensive autologous and PBMC-derived therapies.
Additionally, using a master cell bank eliminates the need for individualized cell collection, simplifying chain-of-identity and reducing chain-of-custody risks. However, iPSC manufacturing poses challenges: iPSCs are anchorage‑dependent and traditionally cultured in 2D, limiting scalability. Recent progress — such as the use of microcarriers that enable suspension (3D) culture — alleviates this concern.50
4. Navigating Regulatory Complexity
The regulatory landscape for cell therapies is complex, with each country imposing distinct clinical development and approval requirements. Early engagement with agencies through initiatives like the FDA’s INTERACT meetings and Europe’s PRIME program, along with harmonization efforts via ICH, provides unified quality and safety benchmarks and helps avert costly delays through early regulatory consultancy.51-53 Strict adherence to GMP-grade facilities and comprehensive CMC documentation poses hurdles for PBMC-derived therapies. Autologous products face challenges in standardizing individualized processes and documenting critical quality attributes, while allogeneic products suffer from donor variability, inconsistent batch performance, limited testing, and gaps in protocols — issues that often lead to clinical holds.54,55
In contrast, allogeneic iPSC‑derived cell therapies rely on a well‑characterized master cell bank from a single healthy donor, which eliminates the need for patient-specific collection, simplifies chain-of-custody, and reduces variability. Their scalable, off‑the‑shelf manufacturing enables standardized GMP protocols and consistent product quality, accelerating clinical development.
However, iPSC manufacturing can produce multiple sub‑clones due to genetic and epigenetic alterations, traditionally managed by manual clone selection by human operators, which can be prone to subjective decision making.56 Automating iPSC handling and employing digital twin simulations can optimize workflows and maintain genomic integrity for clinical‑scale production.57,58
Obstacles To iPSCs Reaching Their Full Potential
Despite progress, potential barriers could hinder iPSC-derived cell therapy's success. One major stumbling block is the inherent complexity of iPSC manufacturing. Although iPSC systems offer scalability and multi‐gene editing advantages, challenges remain in transitioning from traditional 2D adherent cultures to scalable suspension or 3D formats — this process could introduce variability in differentiation efficiency and genetic stability among clones.
Furthermore, transitioning to a 3D, end-to-end closed and automated process is still cost-prohibitive, thus limiting broader adoption. Additionally, the potential for genomic mutations and epigenetic alterations during extended culture periods requires robust quality control measures, which can drive up costs and complicate regulatory approval. Regulatory hurdles, particularly in international markets, add complexity to global implementation. High development and treatment costs make reimbursement models difficult to establish.
Additionally, achieving effective and safe therapies for heterogenous tumor populations without antigen escape remains a scientific challenge requiring further breakthroughs.
While the scientific community increasingly recognizes the benefits of iPSC-derived platforms, the broader investment landscape has been slow to catch up. Many investors remain more comfortable with established PBMC-based therapies due to historically greater clinical data and familiarity, which limits capital flow into iPSC technologies and may slow their market adoption.
Conclusion
The journey to transform cell therapy for solid tumors is not just a scientific challenge — it’s a critical evolution in cancer care. The high mortality rates and frequent relapses associated with solid tumors, compounded by the limitations of traditional CAR‑T and CAR‑NK approaches, highlight an urgent need for innovative solutions. iPSC‑derived cell therapies offer a promising path forward by providing scalable, off‑the‑shelf products that overcome many hurdles inherent to PBMC‑derived methods. These include improved gene editing capacity, reduced manufacturing variability, simplified chain‑of‑custody processes, and the potential to lower production costs — all crucial for overcoming an immunosuppressive tumor microenvironment, mitigating antigen escape, and improving treatment accessibility.
By integrating insights on overcoming tumor microenvironment suppression, addressing tumor heterogeneity, and streamlining manufacturing and regulatory strategies, this comprehensive analysis underscores how multidisciplinary approaches are essential to surmount current obstacles. Researchers, clinicians, and investors can leverage these insights to refine cell therapy designs, adopt innovative manufacturing technologies like microcarrier‑based suspension cultures and digital twin simulations, and navigate complex regulatory landscapes more effectively. Ultimately, mastering these challenges not only accelerates the clinical translation of novel therapies but also paves the way for more affordable and broadly accessible cancer treatments, offering renewed hope to patients worldwide.
About The Author:
Shubhranshu Gupta, Ph.D., is a scientist and entrepreneur specializing in cellular immunotherapy, with a focus on CAR-T and CAR-NK cell therapies for hematological and solid tumors. He is the CEO and CSO at a stealth mode biotech startup that he co-founded in 2024, and serves as a scientific advisor for multiple expert insight networks. Dr. Gupta has held research positions at Caribou Biosciences, Fate Therapeutics, and Baylor College of Medicine. His work includes early discovery pipelines, gene editing, and developing cell-based assays, with several publications in peer-reviewed journals. He earned his Ph.D. in the area of immunological memory in cell therapy at Baylor College of Medicine. Contact him on LinkedIn.