Harnessing The Human Virome For The Next Generation Of Gene Therapy Vectors
By Tuyen Ong, MD, MBA, Ring Therapeutics and Flagship Pioneering
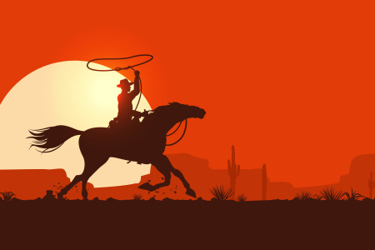
We spend so much time thinking about viruses that cause disease, especially over the last two years, but have you ever considered the fact that not all viruses are harmful? What if we could harness the power of relatively harmless viruses to solve major obstacles in medicine? For the first time ever, researchers are focusing on growing our understanding of a relatively understudied class of harmless viruses that live within all humans and could be the answer to many questions in genetic medicine.
Since its discovery, the human microbiome has served as a rich source of therapeutics and medical insights,1-3 but a lesser-known component of the microbiome, the virome, can potentially offer solutions to problems that face medicine today. The human commensal virome is composed of a plethora of viruses that live harmoniously within all of us.4 These commensal viruses often have broad tropisms, inhabiting a wide range of tissues, such as our eyes, hearts, and livers, all the while subverting the immune response that accompanies foreign viral infections.
Throughout history, researchers have discovered how to transform foreign viruses into life-saving therapies. This began as vaccines in which viral material prepares the immune system to fight a future infection and even led to using viruses to fight certain cancers. But one of the most recent advancements in using foreign viruses to fight human disease is gene therapy — engineering viruses to replace or fix genetic defects. Though as game-changing as these viral-based gene therapies are, many barriers still limit their therapeutic potential.
Gene Therapies: The Costs & Benefits Of Using Viruses To Save Lives
Since the first U.S.-approved therapy for a genetic disease in 2017,5 researchers began ushering in an era of treatment possibilities for those with debilitating genetic diseases using foreign viral vectors.
Viral vectors use the capsid of a virus to encapsulate and deliver genetic cargo to the cell nucleus, and because viruses are so effective at entering host cells, they quickly became standard.6-7
The most common viral vectors in gene therapy today are adeno-associated viruses (AAVs), non-enveloped viruses belonging to the parvovirus family discovered in the 1960s.8-9 At the outset, AAVs were promising, offering a potential delivery vehicle that was tractable and relatively safe in preclinical models. Additionally, the viral biology of AAVs suggested prolonged safety profiles that would largely avoid mutagenesis, a likely result if the genetic material integrated within the host genome. Although some genomic integration has been observed in animal models,10-11 the AAV vector cargo largely remains as an episomal element within the nucleus, a positive in that there’s less risk of insertional mutation but with a complementary downside whereby the cargo is diluted as cells grow and divide. This dilution requires redosing of the gene therapy in any tissue with dividing cells. However, once injected, the formation of neutralizing antibodies against the foreign AAV viral vectors has prevented safe redosing and resulted in the need for large, and often toxic, initial doses to be effective. These toxic doses have at times resulted in tragic outcomes in clinical trials.12-13 Additionally, natural prevalence of neutralizing anti-AAV antibodies in large proportions of the population (>40% in some serotypes14) precludes some patients from receiving even an initial dose of AAV-driven gene therapy.
Lentiviral vectors, a spherical enveloped RNA retrovirus, are another common viral vector in gene therapy today. Lentiviruses elicit a relatively weak immune response in animal models,15-16 and recent engineering successes have enhanced transduction of specific cell types.17 However, they are integrating viral vectors, which can provide long-term transgene expression, but also have a much higher risk of oncogenesis as a result of integration.18
Although viral vectors remain an ideal model for delivering genetic cargo because of their ability to efficiently enter host cells, the immunogenicity of AAVs and other vectors is a significant hurdle. The ideal viral vector platform would not elicit an immune response, would be potent, and would be diverse enough to infect targeted tissue types selectively.
The commensal virome may hold the solution. Recent research has shown how it may solve some of the greatest challenges remaining that are preventing the promise of gene therapy from reaching its full potential as a truly transformative treatment option.
Harnessing The Natural Human Virome To Design Better Vectors
Advances in metagenomic sequencing have revealed insights into the human virome with boundless value for gene therapy researchers. This includes exploring the diversity of a family of small commensal viruses called anelloviruses that have never been associated with disease.19-20
Almost every human maintains a diverse community of anelloviruses, called the anellome, that makes up the largest family of eukaryotic viruses within the human commensal virome.21
Anellovirus genomes do not integrate into the human genome, exhibit a broad yet specific tropism, and do not elicit a robust immune response, which allows them to persist in us for months or even years.19,22 These key facets make anelloviruses a promising new tool in our effort to overcome today’s limitations in genetic medicine.
Anellovirus Discovery & Screening
Anelloviruses are highly prevalent in humans, and many individuals often harbor a unique and dynamic anellovirus landscape.23 Additionally, a recent blood transfusion study utilizing our platform showed that anelloviruses are extremely diverse, highly transmissible, and persistent, with donor anelloviruses detected in recipients more than 200 days post transfusion (the last time point of data collection).23
Not only were anelloviruses shown to be both transmissible and persistent, but evidence also suggests that they are redosable. Despite significant genomic similarity between anelloviruses of recipients and donors, the anelloviruses from both donor and recipient co-exist within the recipient without causing any neutralizing immune response for an extended period of time.23 This critical facet of anellovirus biology could finally permit redosing of a viral vector in gene therapy.
Building on this data, Ring scientists set out to harness the unique anellovirus biology for therapeutic application. For a newly identified anellovirus genome, the team can recreate it in vitro as a fully synthesized recombinant human anellovirus, then recover it using traditional viral purification methods, marking the first time human anelloviruses have been produced in vitro.24
These recombinant anelloviruses can also be vectorized, loading the anellovirus capsid with chosen DNA cargo that can be successfully transduced into human cells in vitro.24 Ring dubs this novel viral vector an Anellovector.
Although natural anelloviruses do not elicit a robust immune response, the question remained whether humans maintain immunity against synthesized Anellovectors. Similar to natural anelloviruses and to no surprise to the Ring team, preclinical studies of its initial Anellovectors identified low preexisting immunity against it in a pool of thousands of human donor sera,24 validating the potential utility of Anellovectors as the next generation of gene therapy vectors.
Unique Anellovector Features With Promising Therapeutic Potential
Anellovectors harness the unique biology of human anelloviruses and have strong therapeutic potential because of five key features:
Redosability
Anelloviruses are ubiquitous within the human body and, having co-evolved with humans for millennia, largely avoid triggering any significant immune reactions. Transfusion of similar anelloviruses between human patients showed persistence without a deleterious immune response.23 Through harnessing the unique feature of immune stealth of anelloviruses, Anellovectors have the potential to treat patients requiring redosing, such as those suffering from spinal muscular atrophy and hemophilia.
Circumvent Preexisting Immunological Barriers
Greater than 40% of patients maintain a natural prevalence of some serotypes of anti-AAV neutralizing antibodies,14 precluding them from receiving even an initial dose of any AAV-driven gene therapy. Because humans maintain low preexisting immunity to anelloviruses and Anellovectors, their use may offer the opportunity for these patients to receive a potentially life-saving gene therapy treatment.
Tropism with Cellular and Tissue Specificity
Many tissue types are elusive to current gene therapy vectors and are deemed “unreachable.” However, anelloviruses exhibit a broad tissue tropism, being found from head to toe of most humans,19 including those that are currently “unreachable.” Therefore, Anellovectors can be designed through harnessing specific anelloviruses that exhibit a targeted tropism. This specificity opens the door for potential solutions to a broad range of genetic diseases across tissue types, including the liver, offering a safer option with no hepatotoxicity.
Lack of Insertional Mutagenesis
Current virus-based gene therapy platforms, including some instances with AAVs, can randomly integrate within the human genome, disrupting the function of important genes and sometimes even causing oncogenesis. As with anelloviruses, Anellovector cargos remain as episomes in the nucleus and do not affect the host genome, minimizing the potential risk and making for a safer form of gene therapy.
Versatility
Because Anellovectors can be synthesized and vectorized, Anellovectors can be engineered to accommodate a wide variety of therapeutic modalities, from single-stranded DNA for gene therapies to potentially enabling delivery of larger DNA products through packaging of mRNA.
Looking To The Future Of Gene Therapy
Through a better understanding of the commensal virome, there’s hope for a brighter future for gene therapy. This promising new technology may help gene therapy reach its full potential by overcoming the current limitations with repeat dosing, toxicity, and limited tropism.
Ring’s research team has uncovered and characterized the world’s largest collection of commensal anelloviruses and is harnessing those best suited for specifically targeting a wide array of diseases with significant unmet needs. The unique biology of anelloviruses may indeed provide a foundation for an unparalleled new gene therapy platform and the resulting new class of genetic medicines.
References
- Integrative, H.M.P., Proctor, L.M., Creasy, H.H., Fettweis, J.M., Lloyd-Price, J., Mahurkar, A., Zhou, W., Buck, G.A., Snyder, M.P., Strauss III, J.F. and Weinstock, G.M., 2019. The integrative human microbiome project. Nature, 569(7758), 641-648.
- Helmink, B.A., Khan, M.W., Hermann, A., Gopalakrishnan, V., and Wargo, J.A., 2019. The microbiome, cancer, and cancer therapy. Nature Medicine, 25(3), 377-388.
- Cully, M., 2019. Microbiome therapeutics go small molecule. Nature Reviews Drug Discovery, 18(8), 569-572.
- Kumata, R., Ito, J., Takahashi, K., Suzuki, T., and Sato, K., 2020. A tissue level atlas of the healthy human virome. BMC Biology, 18, 1-15.
- Chow, V.A., Shadman, M., and Gopal, A.K., 2018. Translating anti-CD19 CAR T-cell therapy into clinical practice for relapsed/refractory diffuse large B-cell lymphoma. Blood, The Journal of the American Society of Hematology, 132(8), 777-781.
- Dunbar, C.E., High, K.A., Joung, J.K., Kohn, D.B., Ozawa, K., and Sadelain, M., 2018. Gene therapy comes of age. Science, 359(6372).
- Mendell, J.R., Al-Zaidy, S.A., Rodino-Klapac, L.R., Goodspeed, K., Gray, S.J., Kay, C.N., Boye, S.L., Boye, S.E., George, L.A., Salabarria, S. and Corti, M., 2020. Current clinical applications of in vivo gene therapy with AAVs. Molecular Therapy, 29(2), 464-488.
- Atchison, R.W., Casto, B.C., and Hammon, W.M., 1965. Adenovirus-associated defective virus particles. Science, 149(3685), 754-755.
- Hoggan, M.D., Blacklow, N.R., and Rowe, W.P., 1966. Studies of small DNA viruses found in various adenovirus preparations: physical, biological, and immunological characteristics. Proceedings of the National Academy of Sciences of the United States of America, 55(6), 1467.
- Hanlon, K.S., Kleinstiver, B.P., Garcia, S.P., Zaborowski, M.P., Volak, A., Spirig, S.E., Muller, A., Sousa, A.A., Tsai, S.Q., Bengtsson, N.E. and Lööv, C., 2019. High levels of AAV vector integration into CRISPR-induced DNA breaks. Nature, 10(1), 1-11.
- Nguyen, G.N., Everett, J.K., Kafle, S., Roche, A.M., Raymond, H.E., Leiby, J., Wood, C., Assenmacher, C.A., Merricks, E.P., Long, C.T. and Kazazian, H.H., 2021. A long-term study of AAV gene therapy in dogs with hemophilia A identifies clonal expansions of transduced liver cells. Nature Biotechnology, 39(1), 47-55.
- Vandamme, C., Adjali, O., and Mingozzi, F., 2017. Unraveling the complex story of immune responses to AAV vectors trial after trial. Human Gene Therapy, 28(11), 1061-1074.
- Wilson, J.M., and Flotte, T.R., 2020. Moving forward after two deaths in a gene therapy trial of myotubular myopathy. Human Gene Therapy, 31(13-14), 695-696.
- Calcedo, R., Vandenberghe, L.H., Gao, G., Lin, J., and Wilson, J.M., 2009. Worldwide epidemiology of neutralizing antibodies to adeno-associated viruses. The Journal of Infectious Diseases, 199(3), 381-390.
- Abordo-Adesida, E., Follenzi, A., Barcia, C., Sciascia, S., Castro, M.G., Naldini, L., and Lowenstein, P. R., 2005. Stability of lentiviral vector-mediated transgene expression in the brain in the presence of systemic antivector immune responses. Human Gene Therapy, 16(6), 741-751.
- Baekelandt, V., Eggermont, K., Michiels, M., Nuttin, B., and Debyser, Z., 2003. Optimized lentiviral vector production and purification procedure prevents immune response after transduction of mouse brain. Gene Therapy, 10(23), 1933-1940.
- MacKenzie, T.C., Kobinger, G.P., Kootstra, N.A., Radu, A., Sena-Esteves, M., Bouchard, S., Wilson, J.M., Verma, I.M. and Flake, A.W., 2002. Efficient transduction of liver and muscle after in utero injection of lentiviral vectors with different pseudotypes. Molecular Therapy, 6(3), 349-358.
- Ciuffi, A., 2008. Mechanisms governing lentivirus integration site selection. Current Gene Therapy 8(6), 419-429.
- Liang, G., and Bushman, F.D., 2021. The human virome: assembly, composition and host interactions. Nature Reviews Microbiology, 1-14.
- Koonin, E.V., Dolja, V.V., and Krupovic, M., 2021. The healthy human virome: From virus–host symbiosis to disease. Current Opinion in Virology, 47, 86-94.
- Tyschik, E.A., Rasskazova, A.S., Degtyareva, A.V., Rebrikov, D.V., and Sukhikh, G.T., 2018. Torque teno virus dynamics during the first year of life. Virology Journal, 15(1), 1-4.
- Kaczorowska, J., and van der Hoek, L., 2020. Human anelloviruses: diverse, omnipresent and commensal members of the virome. FEMS Microbiology Reviews, 44(3), 305-313.
- Arze, C.A., Springer, S., Dudas, G., Patel, S., Bhattacharyya, A., Swaminathan, H., Brugnara, C., Delagrave, S., Ong, T., Kahvejian, A., Echelard, Y., Weinstein, E.G., Hajjar, R.J., Andersen, K.G., and Yozwiak, N.L., 2021. Global genome analysis reveals a vast and dynamic anellovirus landscape within the human virome. Cell Host & Microbe, 29(8): 1305-1315.
- Nawandar, D.M., Arze, C.A., Timpona, J., Boisvert, N., Trivedi, M., Diaz, F., Lebo, K., Prince, C., Scano, C., Springer, S., Bounoutas, G., Fusco, R., Dudas, G., Patel, S., Bhattacharyya, A., Swaminathan, H., Lious, S., Swanson, K., Ong, T., Kahvejian, A., Echelard, Y., Weinstein, E.G., Hajjar, R.J., Anderson, K.G., Delgrave, S., and Yozwiak, N.L., 2021. Anelloviruses are highly diverse, ubiquitous commensal denizens of the human virome, and show promising properties as novel gene delivery vectors. 2021. Human Gene Therapy: ESGCT Collaborative Virutal Congress, October 2021 Abstracts. A1-A152.
About The Author:
Tuyen Ong, MBA, MD, is CEO of Ring Therapeutics and CEO/Partner of Flagship Pioneering. He is a physician and bioentrepreneur. Prior to joining Ring Therapeutics in 2020, he served as senior vice president at Biogen and as Chief Development Officer at Nightstar Therapeutics until its acquisition by Biogen. During this time, he was involved with the company’s public listing on the NASDAQ, corporate and gene therapy strategy, investor, and M&A activities. Ong brings more than 20 years of clinical and drug development experience from both large pharma and biotech, working in the fields of genetics, ophthalmology, and rare disease at PTC Therapeutics Inc., Bausch and Lomb Inc., and Pfizer. He is a member of the Royal College of Ophthalmologists and a Churchill Fellow.