Go From Batch To Continuous RNA Purification With mAb Equipment And QBD
A conversation with Zoltán Kis and Adithya Nair, University of Sheffield
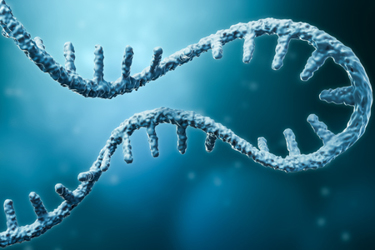
The most accessible mRNA chromatography purification method historically has come in a single flavor — batch mode.
But batch oligo-deoxythymidine (oligo-dT) chromatographic purification of mRNA has drawbacks; among the most notable are wasted product and limited production capacity.
Researchers at the University of Sheffield recently developed a novel method using oligo-dT to purify polyadenylated mRNA continuously, an achievement they say has stunning implications for productivity, efficiency, and costs.
We asked Sheffield’s Zoltán Kis, Ph.D., and Adithya Nair, Ph.D., two co-authors on a paper published in Molecular Therapy Nucleic Acids detailing their research, about the advancement and what it means for mRNA production for research processes up to GMP.
Here’s what they told us. Their answers are edited for brevity and clarity.
Can we start with a brief overview of your research on oligo-deoxythymidine chromatography? This is a well-established technique for mRNA, but you wanted to see how it works using QbD to develop a fully continuous process. Correct?
Kis: Oligo-dT-based purification is an affinity method in which oligo-dT functionalized stationary phase in beads, monolith or membrane format can be used. The important thing is that there are ligands that have oligo-dTs, which bind to the poly(A) tail of the mRNA with hydrogen bonds.
It only captures the poly(A) tail. If an mRNA doesn't have a poly(A), it doesn't get captured. The main question for us was how to make this more efficient, how to make this work in a way that you can purify more per unit time, per unit scale.
In this paper, we described how we applied chromatography. We used the multi-column format where we had four chromatography columns all operating at the same time.
Basically, the way this works is that you have two columns in the loading zone.
The advantage is that you can fully load the first column and what would be wasted actually gets captured in the second column. You reduce waste and maximize column utilization.
So, that's the first two columns. For the two remaining, one is being prepared for loading, called regeneration, and the other one is just being eluted. They all go through the steps of bind-elute.
In the next step, everything shifts. The first column, which was loaded, now gets eluted, and the second and third columns become the loading zone. The fourth column begins regeneration.
You keep repeating these steps, you cycle these columns, and they all work at the same time. The important thing is that you load fully and continuously. You never disrupt the load.
So, each column gets eluted. As I mentioned, the key advantages are that you properly load the column to its high capacity. You utilize all the ligands on all the resin, so you minimize losses because what you would lose, you capture in a second column.
And there's also an interesting thing called a post-load wash. In a single-column format, what you push out after load goes to waste. Here, the post-load wash goes into the fresh column. That’s another way to minimize losses.
Overall, the main advantage is more productivity. If you look at how much material it purifies per unit time, per unit scale. It’s something like 5.75-fold. It’s nearly 600% more productive.
The QbD strategy was used to quantify the impact of critical process parameters (salt concentration in the buffer flow rate during column loading and mRNA concentration), along with critical materials attributes (salt type, on CQAs and KPIs). Once the process was optimized in batch mode, we applied these conditions in a continuous mode of operation.
Overview of the proposed mRNA-based vaccines and therapeutics manufacturing process and the oligo-dT affinity purification method. Source: Zoltán Kis and Adithya Nair. Originally published in Molecular Therapy — Nucleic Acids, Dec. 10, 2024.
Are there other advantages to continuous oligo-dT chromatography compared to the oligo-dT batch method?
Nair: Regardless of the chromatography chemistry, the continuous process would always be more efficient. If you want the same performance, you could do it in a batch mode, but you would need a huge column to purify the same amount of mRNA.
Here, you have four columns working in a loop. This setup has reduced the overall production footprint. It gives you a higher process efficiency so you can deal with more input material without being constrained by space.
Once you reach a steady state in a continuous mode of operation, that's the ideal scenario.
I would say from a chemistry point of view, the mode of unit operation (i.e, batch or continuous) is not relevant because we use a rigorous quality by design framework to develop the process initially from a batch mode and then apply it to the continuous process.
The QbD framework was the main driver here. Could you talk about how you used QbD to engineer this?
Kis: So, we use this similarly to how we optimize other unit operations in the manufacturing process. We applied the quality by design framework. It is a long framework, but one part of it is looking at the CQAs (critical quality attributes), which impact safety and efficacy. You really want to obtain products with CQAs meeting your quality/efficacy requirements. If those are not mapped onto the manufacturing process, you're doing something wrong.
Then, look at key performance indicators or KPIs of the manufacturing process. Those normally relate to things like costs and productivity. You can define them in different ways, but it normally means that you just make things more efficiently at a lower cost.
Additionally, we adjust the CPPs and CMAs, based on the framework to improve the CQAs and KPIs.
Nair: Oligo-dT chromatography itself is not a new unit operation. The only problem is, it was never used before the COVID-19 pandemic at this scale. To develop this unit operation on an industrial scale, you need to understand the process parameters that are influencing your output. The QBD framework is really useful to understand the overall process and how to optimize at scale.
Another advantage of mRNA technology is that it is a platform technology, and the QBD framework complements it very well.
As far as I'm concerned, that would be the best part of following the QBD framework, because it's highly transferable. The information that you get from one set of experiments or one modality can be transferred to another one. That's a huge advantage because you don't have to start from scratch.
Continuous chromatography for other biologic products such as mAbs is more established. Are there any lessons from those that inform continuous oligo-dT chromatography platform development? Where are the similarities?
Kis: It's just another chromatography method. You have a column in which you have some kind of solid support, which has affinity ligands. Here, we have oligo-dT. For proteins, you would have protein A.
You would operate this in bind and elute mode. That means that for each column you would go through the steps of preparing the column — equilibrating the column with the buffers — loading the column, then washing the column, eluting the column, and finally regenerating the column. Obviously, buffers are different, what you load is different, and the column is different, but the concept is the same.
Nair: There was no equipment that could handle continuous chromatography for mRNA when we started this whole thing. Initially, all the equipment was designed for protein purification. It was never meant for mRNA purification.
Again, that's where the whole QBD framework helps our cause because the underlying principle itself is similar. You understand the process parameters and you see how it affects the quality attributes that you want to maintain or that you are interested in. We are further refining the QbD framework and applying it to support our mRNA production process development work.
What might hinder an mRNA developer from implementing this method commercially at scale?
Kis: There are multiple columns involved, there are multiple valves, there are multiple things happening. Overall, it’s more complex to operate and generally more complex to take to GMP, because there are multiple things you have to validate. I would say it's not impossible, it's just more challenging.
On the other hand, because you have a lot more efficiency, you might not need to always scale, depending on what you're talking about. If there isn’t a pandemic, you might not need to scale it that much.
Now, we’re developing equipment that will allow GMP manufacturing. All the equipment we used for purification was borrowed from proteins — chromatography, TFF (tangential flow filters) — just the closest we could find. It was good enough, but never really optimized for mRNA. We’re working with a local engineering company that does equipment design, the hardware, electronics, and the control software to build equipment able to do the enzymatic synthesis of RNA, purification, LNP formulation and more purification after LNP formulation in one piece of equipment. It’s like a push-button solution for making RNA from drug substance all the way to drug product.
Nair: A bottleneck there, regardless of which unit operation you're talking about, is a lack of process analytical technology. mRNA manufacturing at this scale is very new. It's still maturing from a GMP or from an advanced manufacturing point of view.
The unit operation is dependent on mRNA transcripts with a poly(A) tail. This purification technique might not be useful for products where the poly(A) tail is not required. Next-generation mRNA drug substances like self-amplifying mRNA (saRNA) and circular RNA (circRNA) will be more challenging to purify with this unit operation (especially at commercial scale).
If you give it enough time, I'm pretty sure continuous mRNA manufacturing is going to reach there. Like mAbs or any other modality, it might take a few more years, but it’ll definitely get there.
Editor’s note: Zoltán Kis and Adithya Nair wish to acknowledge the other authors from their research group who contributed to their research, including lead author Jixin Qu and co-authors George Muir, Kate Loveday, Zidi Yang, Ehsan Nourafkan, Emma Welbourne, Mabrouka Maamra, and Mark Dickman.
About The Experts:
Zoltán Kis is a senior lecturer (associate professor) at the School of Chemical, Materials and Biological Engineering at The University of Sheffield and an honorary lecturer at the Department of Chemical Engineering, Imperial College London. He leads a multidisciplinary team that is innovating and digitalizing RNA vaccine and therapeutics production platform technologies. His work is addressing the challenges of producing large volumes of RNA-based vaccines and therapeutics, rapidly, at high quality and at low cost in a disease-agnostic manner. He has previously worked as a research associate in the Future Vaccine Manufacturing Hub at Imperial College London. He obtained his Ph.D. in bioengineering from Imperial College London, U.K., and holds an M.Sc. in applied biotechnology and a B.Eng. in chemical with biochemical engineering.
Adithya Nair is a post-doctoral research associate within the UK-SEA Vax Hub at the University of Sheffield. His work focuses on techno-economic modeling and process development for mRNA-based product manufacturing. He obtained his doctoral degree from Osaka University, Japan, and holds a master’s degree in industrial biotechnology.