Gene Therapy Competitive Dynamics: Winner Takes All?
By Clara Zacharko, Andrew Parece, and Matthew Majewski
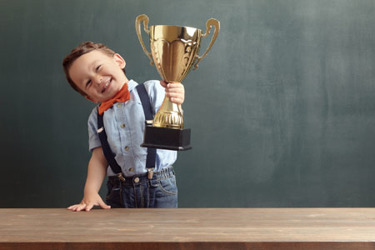
With many gene therapies in development for an increasing number of indications, their curative promise presents a unique competitive dynamic as they enter the market over the coming years. A one-time treatment with the potential to cure patients could create a “winner takes all” dynamic, whereby second and later entrants for the same indication would have significantly smaller patient populations, such as treatment-resistant or inappropriate patient segments. In this scenario, competitive analysis in the field of gene therapy becomes crucial to assess the market landscape and identify potential opportunities and challenges for gene therapy developers.
The extent to which curative gene therapies will create such a “winner takes all” dynamic will depend on how similar gene therapy treatments are considered by payers, key opinion leaders (KOLs), and physicians to be comparable. Or, alternatively, when do differences between therapies within an indication become large enough to require payers to cover both products instead of choosing a preferred product? Furthermore, the timing of gene therapy approval and the number of products launching imminently will also influence the opportunity for payers to select a preferred agent and options for “warehoused” patients who have been holding out in expectation of superior therapies. In Figure 1, we present a framework illustrating the effect of timing, differentiation, and the number of products within a given indication on the likelihood of payers selecting a preferred agent. The ensuing discussion provides supporting rationale for the competitive outcome of each scenario by evaluating variations in technology, durability, and safety for new gene therapies. Gene therapy differentiation plays a crucial role in determining how these therapies are perceived and adopted in the market.
Figure 1: Illustrative scenarios affecting competitive outcomes for new gene therapies.
Source: CRA analysis
What Are The Issues That Will Matter For Product Differentiation?
Vector Technology
The genetic material within a gene therapy is introduced to a patient’s cell through a carrier, most often in the form of a modified viral vector. The ideal viral vector will be both immune-silent, not triggering an immune response in the patient, and efficient in its delivery, specifically targeting the appropriate cells or tissue.1 These two key traits are complementary – the more specific the targeting ability of the viral vector, the lower the dose of the therapy required, and the less likely an immune response will be triggered in the patient. The targeted gene and traits of the transgene product, such as size, within a specific indication, will affect the choice of the vector.2
Durability
There is a risk for gene therapies over whether they will be curative across a patient’s entire lifetime. Not only does this uncertainty around durability affect the commercialization process, but it also raises questions of safety if patients require re-dosing and the subsequent risk of further side effects, such as immune activation. Once the viral vector has been introduced to the patient, antibodies will be developed by the patient’s immune system, making repeat treatment or dosing with the same viral vector challenging or impossible.3 Durability of novel gene therapies is also a key payer concern. Even for therapies that capture a large “winner takes all” patient population, there may be a subset of patients that do not respond or don’t experience a durable cure. Given the relatively high price of these therapies, payers face risks of additional treatment costs and are exploring risk-sharing warranties, payment options (annuities), and outcomes-based agreements to mitigate these risks
Safety
Gene therapies raise safety concerns around immune-triggering vectors, as well as general immunotoxicities resulting from the viral vector, impurities in the product, and effects from the transgene product.4 Dorsal root ganglia (DRG) toxicity is also a concern for gene therapies that are administered directly into the spinal cord fluid; future therapies will need to address these concerns around toxicity and associated morbidity.5 General safety, from high standards in manufacturing to short- and long-term adverse effects in patients, is a growing concern with the commercialization of gene therapies. For example, in the U.S. the FDA has started to request that companies include risk mitigation strategies in their submissions for regulatory approval6 and has raised the standard of manufacturing and production quality because of the increase in the number of gene therapies in clinical development.7
How Does Gene Therapy Differentiation Vary By Therapy Area?
When considering issues that matter for gene therapy differentiation, nuances become apparent within specific indications (see Figure 2). Most gene therapies are being developed in rare disease and oncology categories. An indication caused by or linked to a monogenic mutation is an ideal candidate for gene therapy because it is caused by a mutation or error within a single gene, which can be targeted by the therapy. This section assesses the opportunity for differentiation in four specific monogenic rare disorders for which gene therapies are being developed: Duchenne muscular dystrophy (DMD), hemophilia A, hemophilia B, and inherited retinal disease (IRD).
Figure 2: Gene therapy traits impacting product differentiation by indication.
Source: CRA analysis
Duchenne Muscular Dystrophy (DMD)
DMD is the most common childhood onset form of muscular dystrophy. The disease affects males almost exclusively and symptoms tend to appear between the ages of 3 and 5. The estimated birth prevalence of DMD is 1 in every 3,500 live male births.8 There is currently no cure for DMD but there are three FDA-approved non-gene therapy medications: Emflaza (PTC Therapeutics), Exondys 51 (Sarepta Therapeutics), and Vyondys 53 (Sarepta Therapeutics). Current non-gene therapy DMD treatments are focused on managing the symptoms and related complications of the illness. The standard of care for DMD is glucocorticosteroids, which only slow down disease progression.9,10
The DMD gene, which has 79 exons, is the largest gene in the human genome.11 Mutations in the genes, which cause one or more of the exons to be missing, result in the absence of the dystrophin protein. However, due to the large size of the gene for dystrophin, viral vectors used in gene therapies for DMD are only able to deliver mini or micro versions of the gene, selected for containing important functional aspects of the gene.12 This may create heterogeneity across gene therapies for DMD and challenges in identifying the correct patient population for which a given mini or micro gene will provide an effective cure. It may also provide an opportunity for multiple gene therapies to be viable in DMD to treat different patient segments.
At least 14 gene therapies are in development for DMD at various phases (ranging from research projects to Phase 3 trials) from manufacturers including Pfizer, Sarepta Therapeutics, Astellas Gene Therapies, and Regenex Bio (see Figure 3). Most gene therapies in development use different adeno-associated viral (AAV) vectors and have different versions of the mini or micro dystrophin gene that is delivered. Some manufacturers, such as Sarepta Therapeutics, are developing multiple gene therapies for DMD.13
DMD is a condition for which multiple gene therapies may emerge for different DMD patient sub-populations. In this case, a “winner takes all” dynamic is unlikely to develop. Gene therapy differentiation is important in this scenario to address the diverse needs of DMD patients.
Figure 3: Number of gene therapies in development by indication.
Source: EvaluatePharma, 2022
Hemophilia
Hemophilia is a rare genetic disease affecting patients who lack the gene for proteins that are required for normal blood clotting, Factor VIII (hemophilia A) and Factor IX (hemophilia B). The current U.S. prevalence is ~20,000 across hemophilia A and B, with hemophilia A approximately six times more prevalent than hemophilia B. The standard treatment for hemophilia A and B is factor replacement therapy, often administered prophylactically via regular infusions. Up to 30% of patients treated for hemophilia may develop inhibitors in response to factor therapy, rendering this treatment ineffective, although less frequently in hemophilia B patients.14
Hemophilia A and B have a high unmet need, are monogenic disorders, and offer easily measurable endpoints, such as factor levels, making these diseases appealing candidates for gene therapies.15 Currently, at least 16 gene therapies are in development for hemophilia A and at least 12 for hemophilia B, at various phases (see Figure 3). Like DMD, the gene for hemophilia A must be truncated to fit into the viral vector, whereas the gene for hemophilia B is much smaller, allowing it to be inserted into many different vectors, with additional availability for other regulating elements for gene expression to be incorporated.16 Furthermore, durability concerns exist for some hemophilia A gene therapies, whereby patients have experienced the loss of Factor VIII expression and breakthrough bleeding in clinical trials.17
Hemophilia, like DMD, is also a condition for which multiple gene therapies may emerge for different patient sub-populations due to gene therapy differentiation. However, with differences in gene size and respective limitations of therapies, a “winner takes all” dynamic is more likely to develop for hemophilia B than it is for hemophilia A.
Inherited Retinal Disease (IRD)
IRDs are a group of several different genetic disorders that cause vision impairment and, when left untreated, can lead to blindness.18 There are over 27 genetic mutations known to cause IRD, with few effective treatments and some forms not yet treatable.19 The estimated prevalence of all monogenic IRDs is 1 in 2,000, affecting a total of more than 2 million people globally.20 In addition to its monogenic nature, IRDs are prime targets for gene therapy as the eye is an immune-privileged organ and the systemic immune system is not usually triggered in response to foreign antigens in the eye. The systemic adverse events typically associated with viral vector treatments are significantly lower when locally administered, due to the blood-retina barrier, and most known side effects are associated with the injection procedure itself.21 Furthermore, small doses of the viral vector delivering the transgene product are required to target the appropriate tissue.22 While some genes for IRDs are also too large to fit into the AAV vectors, other viral vectors that have a larger capacity, such as lentiviruses (LVs), are candidates and are being evaluated for use.23
With these advantages for IRD gene therapy, the first gene therapy to be approved by the FDA was for Luxturna (voretigene neparvovec) in 2017. Luxturna is indicated for the treatment of an IRD caused by an RPE65 mutation, which affects approximately 1,000 to 2,000 people living in the U.S.24 The RPE65 gene fits into the AAV vector, which Luxturna uses to deliver a functional copy of the gene through a subretinal injection.25 Studies assessing the durability of Luxturna find improvements in retinal sensitivity that remain stable or decline after peaking six to 12 months post-therapy. The manufacturer, Spark Therapeutics, is conducting ongoing trials assessing durability and has entered into outcome-based agreements with some U.S. payers to address concerns about the treatment wearing off, indicating payers may seek to manage first-to-market products.26,27 While there are at least 25 gene therapies in clinical trials for IRD (see Figure 3), each typically targets a specific gene mutation, suggesting that for every distinct monogenic mutation causing IRD, there could be a “winner takes all” gene therapy.28
IRD is also a condition for which sub-populations will emerge, due to differences in the mutated gene. For each mutation identified, it is likely that a “winner takes all” dynamic will occur to treat these monogenic mutations due to immune and tissue targeting advantages specific to the ophthalmological area. By offering unique advantages in terms of efficacy, safety, and long-term durability, differentiated gene therapies can position themselves as the preferred treatment option for specific mutations within the IRD patient population.
Implications For Payer Preferences
With several therapies in the pipeline for both DMD and hemophilia A, the extent to which one of these therapies will generate a “winner takes all” dynamic remains unclear. There are many factors affecting patient, physician, and payer perceptions of gene therapy, from safety and efficacy to cost and durability, and each product in development will demonstrate variations in these outcomes following the completion of clinical trials.29 The extent to which each product will be highly differentiated in these outcomes will depend on the targeted gene and viral vector technology selected. Timing of regulatory approval and product launch will matter; a first-to-market product is more likely to achieve a “winner takes all” dynamic, especially in indications for which there is less gene therapy differentiation, such as a specific IRD mutation and, possibly, hemophilia B.
As new treatments come to market with the potential for heterogeneous efficacy, durability, and safety within a patient population, payers may be hesitant to select preferred agents initially. Without the long-term outlook on the curative ability of such treatments, payers may not select preferred gene therapies should it turn out the effects were not as complete, long-lasting, or safe as thought to be. In these situations, a “winner takes all” dynamic is less likely to emerge.
If payers become more comfortable with treatments available within the gene therapy class and gather additional long-term, real-world data, there may be more willingness to select an exclusive gene therapy, especially if there is limited differentiation for a specific patient sub-type. Gene therapy differentiation becomes increasingly significant in such scenarios. By choosing preferred gene therapies, payers will benefit from receiving lower prices in the short term. However, by preferring certain therapies, payers may disincentivize future entry. Over the long term, this may lead to a reduction in gene therapy R&D as fewer players choose to enter a market where a “winner” has already been identified.
- Bulcha, J. T., Wang, Y., Ma, H., Tai, P. W., & Gao, G. (2021). Viral vector platforms within the gene therapy landscape. Signal Transduction and Targeted Therapy, 6(1), 1-24. Available from: https://www.nature.com/articles/s41392-021-00487-6.
- Sinclair A, Islam S, Jones S. Gene Therapy: An Overview of Approved and Pipeline Technologies. 2018 Mar 1. In: CADTH Issues in Emerging Health Technologies. Ottawa (ON): Canadian Agency for Drugs and Technologies in Health; 2016-. 171. Available from: https://www.ncbi.nlm.nih.gov/books/NBK538378/.
- Shirley, J. L., de Jong, Y. P., Terhorst, C., & Herzog, R. W. (2020). Immune responses to viral gene therapy vectors. Molecular Therapy, 28(3), 709-722. Available from: https://www.cell.com/molecular-therapy-family/molecular-therapy/fulltext/S1525-0016(20)30002-2#.
- Shirley, J. L., de Jong, Y. P., Terhorst, C., & Herzog, R. W. (2020). Immune responses to viral gene therapy vectors. Molecular Therapy, 28(3), 709-722. Available from: https://www.cell.com/molecular-therapy-family/molecular-therapy/fulltext/S1525-0016(20)30002-2#.
- Hordeaux, J., et al. (2020). MicroRNA-mediated inhibition of transgene expression reduces dorsal root ganglion toxicity by AAV vectors in primates. Science Translational Medicine, 12(569). Available from: https://www.science.org/doi/10.1126/scitranslmed.aba9188?rss=1&.
- Fidler, B. (2021). FDA unexpectedly grounds a gene therapy for a rare heart disease. BioPharma Dive. Available from: https://www.biopharmadive.com/news/rocket-fda-clinical-hold-danon-gene-therapy/599941/.
- Gardner, J. (2020, Nov 4) FDA gene therapy holdups suggest closer scrutiny by agency. BioPharm Dive. Available from: https://www.biopharmadive.com/news/fda-gene-therapy-manufacturing-delays-scrutiny/588382/.
- Duchenne Muscular Dystrophy. National Organization for Rare Disorders (NORD). Available from: https://rarediseases.org/rare-diseases/duchenne-muscular-dystrophy/.
- Duchenne Muscular Dystrophy. Genetic and Rare Diseases Information Center (GARD). Available from: https://rarediseases.info.nih.gov/diseases/6291/duchenne-muscular-dystrophy.
- McDonald, C. M., et al. (2018). Long-term effects of glucocorticoids on function, quality of life, and survival in patients with Duchenne muscular dystrophy: a prospective cohort study. The Lancet, 391(10119), 451-461. Available from: https://www.thelancet.com/journals/lancet/article/PIIS0140-6736(17)32160-8/fulltext.
- Muscular Dystrophy News Today (2021). Exon Skipping for Duchenne Muscular Dystrophy. Available from: https://musculardystrophynews.com/exon-skipping-for-duchenne-muscular-dystrophy/.
- Figueiredo, M. (2021) Exon Skipping Dominates DMD Treatment, But What’s on Horizon? Muscular Dystrophy News Today. Available from: https://musculardystrophynews.com/2021/02/25/exon-skipping-dominates-duchenne-gene-therapy-horizon/
- Burke, C. (2020). The Time for DMD Gene Therapy is Now: A Chat with the MDA. BioSpace. Available from: https://www.biospace.com/article/the-time-for-dmd-gene-therapy-is-now-a-chat-with-the-mda/.
- Perrin, G. Q., Herzog, R. W., & Markusic, D. M. (2019). Update on clinical gene therapy for hemophilia. Blood, The Journal of the American Society of Hematology, 133(5), 407-414. Available from: https://www.ncbi.nlm.nih.gov/pmc/articles/PMC6356985/
- Batty, P., & Lillicrap, D. (2019). Advances and challenges for hemophilia gene therapy. Human molecular genetics, 28(R1), R95-R101. Available from: https://academic.oup.com/hmg/article/28/R1/R95/5537029
- Murphy, S. L., & High, K. A. (2008). Gene therapy for haemophilia. British journal of haematology, 140(5), 479-487. Available from: https://www.ncbi.nlm.nih.gov/pmc/articles/PMC2408641/
- Sheridan, C. (2020). A reprieve from hemophilia A, but for how long?. Nature Biotechnology, 38(10), 1107-1109. Available from: https://www.nature.com/articles/s41587-020-0693-y.epdf?no_publisher_access=1&r3_referer=nature&proof=t%C2%A0
- Daiger, S.P., Sullivan, L.S., Bowne, S.J. (2021). Retinal Information Network (RetNet). Available from: https://sph.uth.edu/retnet/.
- Prevent Blindness (2021). Available from: https://preventblindness.org/wp-content/uploads/2021/05/Inherited-Retinal-Diseases-Factsheet.pdf.
- Chen, TC., Huang, DS., Lin, CW. et al. (2021). Genetic characteristics and epidemiology of inherited retinal degeneration in Taiwan. npj Genom. Med. 6, 16. Available from: https://doi.org/10.1038/s41525-021-00180-1.
- Gordon, K., Del Medico, A., Sander, I., Kumar, A., Hamad, B. (2019). Nature Reviews Drug Discovery 18, 415-416. Available from: https://doi.org/10.1038/d41573-018-00016-1.
- Vázquez-Domínguez, I., Garanto, A., & Collin, R. (2019). Molecular Therapies for Inherited Retinal Diseases-Current Standing, Opportunities and Challenges. Genes, 10(9), 654. Available from: https://doi.org/10.3390/genes10090654.
- Ziccardi, L., Cordeddu, V., Gaddini, L., Matteucci, A., Parravano, M., Malchiodi-Albedi, F., & Varano, M. (2019). Gene Therapy in Retinal Dystrophies. International journal of molecular sciences, 20(22), 5722. Available from: https://doi.org/10.3390/ijms20225722.
- FDA News Release (2017). FDA approves novel gene therapy to treat patients with a rare form of inherited vision loss. Available from: https://www.fda.gov/news-events/press-announcements/fda-approves-novel-gene-therapy-treat-patients-rare-form-inherited-vision-loss.
- Spark Therapeutics (2020). Luxturna Mechanism of Action. Available from: https://luxturnahcp.com/how-LUXTURNA-works/mechanism-of-action/.
- Ziccardi, L., Cordeddu, V., Gaddini, L., Matteucci, A., Parravano, M., Malchiodi-Albedi, F., & Varano, M. (2019). Gene Therapy in Retinal Dystrophies. International journal of molecular sciences, 20(22), 5722. Available from: https://doi.org/10.3390/ijms20225722.
- Cohen, J. (2020). Though Promising, Gene Therapies Face Durability and Reimbursement Hurdles. Forbes. Available from: https://www.forbes.com/sites/joshuacohen/2020/12/11/though-promising-gene-therapies-face-durability-and-reimbursement-headwinds/?sh=3d149ddb4f86.
- Leroy, B.P., Pennesi, M.E., Ohnsman, C.M. (2018). Brave New World: Gene Therapy for Inherited Retinal Disease. American Academy of Ophthalmology. Available from: https://www.aao.org/assets/bebfbaef-a092-45b0-9883-c563331546ae/636649294795430000/july-2018-eyenet-supplement-pdf?inline=1.
- Wexler MS, M. (2021). Patients’ Priorities for Gene Therapy: Durability and Bleeding Control. Hemophilia News Today. Available from: https://hemophilianewstoday.com/2021/07/28/hemophilia-patients-gene-therapy-goals-durability-bleeding-control-us-survey/.
About The Authors:
Clara Zacharko is a consulting associate in the Life Sciences Practice at Charles River Associates (CRA). Clara works across strategy and policy consulting with experience in North American and EU markets.
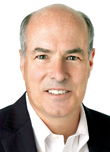
Andrew Parece is vice president in the Life Sciences Practice at Charles River Associates (CRA), a global consulting firm. He has more than 30 years of consulting experience, and specializes in competitive strategy, pricing, quantitative analysis, and market research in the pharmaceutical, biotech, and life sciences industries. He has managed competitive assessments and product launch strategy engagements for several high-profile products and franchises.
Matthew Majewski is vice president in the Life Sciences Practice at Charles River Associates (CRA) and has more than 15 years of experience. He has led a range of engagements from product-related topics such as positioning, pricing, contracting, and navigating disruptive market events to corporate challenges such as channel leadership development, portfolio value creation, and organizational restructuring.