Designing The Cell Therapy Facility Of The Future
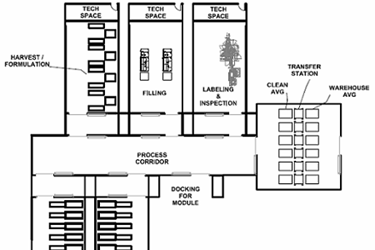
By Herman Bozenhardt, Bozenhardt Consulting Services, and Erich Bozenhardt, IPS-Integrated Project Services
In 1931, Swiss doctor Paul Niehans injected a preparation of live cells from a parathyroid gland into a dying patient. The patient subsequently recovered, and Dr. Niehans had a eureka moment — that injections of living cells can have tremendous therapeutic value.
Today, there are 13 FDA-approved cellular therapies on the market and over 10,000 clinical trials open for cell therapies. (By comparison, there are approximately 3,000 trials are open for antibodies.) Additionally, the FDA just this month created the new Regenerative Advanced Therapy designation to expedite the development and review of cell therapies for serious or life-threatening conditions. The small number of approved products, large number of trials, and favorable regulatory climate raise the odds of a large gap in manufacturing capacity occurring in the not-so-distant future.
As new cell therapies come to market over the next few years, the industry will need to mobilize rapidly and build commercial production facilities that are dedicated to these therapies yet flexible enough to adapt to evolving needs. Driven by process economics, cell therapies present a unique set of criteria for facility design; needs that vary significantly with factors like the type of therapy, patient population, and process closure.
Autologous Or Allogeneic? Distinct — And Common — Challenges
In the cell therapy process space, the low hanging fruit is in allogeneic cell therapies, which involve harvesting cells from a healthy donor (or donors) and transplanting them into others. Allogeneic therapy production can leverage the development efforts vaccine manufacturers have invested in optimizing adherent cell culture designs. The challenges of handling large numbers of 2D bioreactors and automation of processes have already been addressed by various technology suppliers. For example, the Nunc Cell Factory is a commercial offering from Thermo Fisher Scientific that is already used in several vaccine processes and is fully automated.
For allogeneic processes that rely on manual operations, incubators and biosafety cabinets will be involved. However, manual production operations are not sustainable. Advances in technology will drive the incorporation of robots and “lights out”/human-less modularized factories in the long term, though expanding and improving offerings upon an automated, modular backbone is realizable in the immediate future.
In addition, small, boutique fillers are available from multiple vendors to meet the needs of small batches. With these batch sizes, comparisons should be done to determine if the increased variable cost of closed filled vials outweighs the fixed costs of traditional component preparation.
Autologous therapies — where the patient’s own cells are removed, often treated, and later reintroduced to the donor — require an entirely different process/logistical model. Production of treatments for individual patients may be better suited to more of a pharmacy or doctor environment, because many of these therapies are regionally hosted and time sensitive. This need will drive new execution models. One approach is for the process to be executed via an automated, regulated medical device used by a clinician. Fenwall produces an apheresis device targeted at mononuclear cell collection, and Miltenyi Biotec’s Prodigy system provides automated closed culture and separation in a bench top unit.
Figure 1: Automated systems like Miltenyi Biotec’s Prodigy could eventually enable autologous cell therapy production at or near the point of care.
It is possible that the autologous cell therapy facility of the future will be housed within a blood bank. Although they are already FDA regulated, most blood banks would have a steep learning curve to produce cell therapies, since they currently lack the necessary technology and skilled workforce.
Another option for cell therapy providers that want to maintain control of the manufacturing process would be regional manufacturing centers close-coupled with regional hospital systems. This implementation would rely on standardized prefabricated and preconfigured modular cleanrooms to deploy distributed manufacturing centers in a cost-effective and compliant manner. One final facility model would be a one-stop shop, cellular concept housing both collection and production facilities.
A common hurdle with both autologous and allogenic therapies is cold chain shipment, which is time-consuming, expensive, and difficult to validate, given the inherent variables and unknowns associated with carriers, weather, etc. Labeling and then reading those labels on cryopreserved vials is another issue cell banks have had to address and requires a new supply chain model from filling the vial all the way through clinical use. The current approach might drive autologous cell therapy operations to conjoin labeling and secondary packaging with filling, whereas allogenic operations may require a more universal primary label close-coupled to filling and secondary packaging, then handed off to a specialized third party.
Both allogenic and autologous products also require attached cell culture conditions, typically on 2D bioreactors.
What Will Cell Therapy Facilities Of The Future Look Like?
The future facility for this process world will resemble a fishbone from an aerial view. The design and layout will focus on accommodating small articulating robots within the manufacturing area and a more traditional automatic guided vehicle (AGV) traveling up and down the spine. It will have the following design features:
- Central spine: An AGV will travel back and forth down the spine, pushing “cell factories” and nested vials in and out of small operational suites. The AGV will handle wrapped presterilized supplies, components, raw materials, and filled product. This spine will be EU CNC (controlled not classified) grade and have enough width-based room for the AGV and a human mechanic, when needed. The terminal ends of the spine will be AGV docking airlocks that allow the AGV to recharge and undergo preventative maintenance, isolated from the corridor.
- Operating cells: Attached to spine will be various operating cells that have pre-planned process blocks within them. These process blocks/manufacturing cells are built in a modular fashion off-site, and then the equipment is installed, commissioned, and validated at the cell therapy facility. After validation, these cells will be connected to the spine, and the AGV will be programmed to service each cell. Each operating cell will have a specific function to perform, such as cell growth, cell harvest, filling, or packaging. All cells will be autonomous and fully automated, with no accommodation for a human operator. The connection to the spine will be a double-door airlock, to which the modular cells will mate.
Figure 2: “Fishbone” style facility layout, with a central spine (process corridor) and modular operating cells
- Recipes: Production “recipes” will trigger operations within the cell, and required materials will be provided via the AGV.
- Cameras: Remotely monitored cameras will oversee the activities within the cells and assure the recipe is being executed properly.
- Maintenance access: For basic maintenance on the cell equipment, technicians will accessed the cell via the end opposite the AGV. Any major equipment upgrades will be executed by closing the airlock doors at the spine connection and withdrawing the manufacturing pod from the spine. The withdrawn pod will be moved (via wheels or air bearings) to a “turnaround” station for decontamination, human intervention, and retooling.
- Expansion: Manufacturing capacity will be increased or broadened by adding additional cells/pods to the spine and upgrading the AGV’s software with additional recipes.
- Flexibility: The interior of the spine and manufacturing cells will be built with polymer-coated aluminum walls, floors, and ceilings, which will be flexible enough for reconfiguration and repurposing as needed.
- HVAC: The processing cells that handle the manufacturing will have their own autonomous HVAC system that can be made to accommodate any EU HVAC grade. However, since most processes will be self-contained and disposable, the HVAC grade will most likely be CNC or D. The HVAC systems will contain small air handling units (AHUs) mounted on the top of the module. The module interiors and AHU plenums will be fitted with vapor dispensing nozzles to be connected to a sterilizing gas system (VHP, iHP, ClO2, or NO2) for decontamination of a module interior before and after service.
- Utilities: Traditional utilities currently associated with the biotech industry will be minimized, and the use of disposable systems will dominate machines and modules. Small quantities of water for injection (WFI) or cleaning agents will be supplied by the AGV. Gases such as carbon dioxide and clean dry air will be provided by the module into the cellular equipment.
Ultimately, cell therapy manufacturing plants could more closely resemble electronics or precision fabrication plants than what we currently recognize as a biotech plant. However, this concept is necessary to accommodate the tedious level of manipulation, to provide a predictable and reliable manufacturing platform, and to minimize the cost of the facility — and ultimately the cost to the patient.
Conclusion
The large pipeline and high potential therapeutic value of cell therapy products will soon send the industry scrambling for manufacturing solutions and capacity. Dedicated cell therapy facilities constructed to meet this demand will, by necessity, look very different from current biotech facilities, to meet a very unique set of process requirements and business practices. The variety of processes and clinical administration will drive manufacturers and engineers to find novel solutions. The real key to success will be reducing the level of human handling and the associated spatial requirements.
When that time comes, we will look to take the current thinking regarding traditional healthcare, regional manufacturing models, and biotechnology production and turn them on their head — taking a fresh approach, mixing and matching what works to arrive at a new model that leverages the efficiencies of evolving science and an evolving economy.
About The Authors
Herman Bozenhardt has 40 years of experience in pharmaceutical, biotechnology, and medical device manufacturing, engineering, and compliance. He is a recognized expert in the area of aseptic filling facilities and systems and has extensive experience in the manufacture of therapeutic biologicals and vaccines. His current consulting work focuses on the areas of aseptic systems, biological manufacturing, and automation/computer systems. He has a B.S. in chemical engineering and an M.S. in system engineering, both from the Polytechnic Institute of Brooklyn.
Erich Bozenhardt is the process manager for IPS-Integrated Project Services’ process group in Raleigh, NC. He has 10 years of experience in the biotechnology and aseptic processing business and has led several biological manufacturing projects, including cell therapies, mammalian cell culture, and novel delivery systems. He has a B.S. in chemical engineering and an MBA, both from theUniversity of Delaware.