Biocontainment: An Introduction To Control Levels & Practical Design Concepts
By Herman F. Bozenhardt and Erich H. Bozenhardt
In part 1 of our article on biocontainment, we discussed the history of biocontainment as an outgrowth of medical research, especially the effort to develop vaccines for various worldwide diseases. We provided some definition of the biosafety levels (BSLs) established by the Centers for Disease Control and Prevention (CDC) based on risk considerations. We also discussed the regulatory regime for biological agents and, specifically, for designing a facility for an FDA or EU regulated product within the confines of the safety practices dictated by the CDC and design requirements of the NIH.
Our discussion continues with the physical application of GMP design practices as influenced by the CDC and NIH regulations. The first part of our design discussion covers various processes and their applicable BSL levels, to understand the risk levels and what types of processes must be contained. Finally, we will provide some practical (and required) design and building concepts that must be integrated into the GMP process to satisfy the FDA and EU, while taking into account requirements from the NIH and CDC.
Containment Controls By Level
BSL-1
For biosafety level 1, the practices and barriers are in line with GMP expectations for commercial manufacturing. Biosafety cabinets are not required at this level. State or other local codes will not typically allow discharge of live genetically engineered organisms.
Typically, a commercial GMP facility will meet the requirements of BSL-1, and single-use systems are amenable to these processes.
An example of a BSL-1 process is a gene therapy process using a recombinant adeno-associated virus. Because the starting bank and final product are infectious, the whole process is BSL-1. Due to the low requirements for BSL-1, GMP requirements will govern the manipulations used in this process. The low safety concern and low throughput make this process a good opportunity for using a waste management company to decontaminate all the waste materials off-site, thus reducing the facility’s footprint.
BSL-2
Biosafety level 2 requires primary barriers and begins to control flows in and out of spaces. Any open process that might generate an aerosol or splashing must be performed in biosafety cabinets or other physical containment apparatus. The facility needs the following:
- liquid disinfect traps on vacuum lines
- a method of decontaminating waste
- handwashing sink and an eyewash station
- removal of protective clothing/lab coat on leaving the BSL-2 area
- airflow into the suite is recommended, providing a negative pressure
The vast majority of vaccine processes and therapies that use blood or primary cells fall into this category. While the regulations don’t require air locks or directional airflow, typically these features are added for GMP cross-contamination reasons, as most processes have steps where the bulk substance is no longer infectious. Viral screening and inactivation can be used to reduce the portion of the process that is BSL-2. These facilities typically have dedicated bio-waste drains and inactivation systems. In some cases, cell therapy facilities have been able to use certified medical waste haulers (which incinerate the waste) because the bulk of the contaminated waste is solid material.
In general, the industry has adapted single-use systems with a risk assessment based on thorough supplier vetting and pre-use integrity checks. Minor changes in tubing set construction (e.g., going with molded fittings over zip-tied barb fittings) have reduced the risk of leaks to an acceptable level.
An example of a BSL-2 process is the production of a seasonal influenza vaccine from the virus cell bank through virus attenuation. These facilities typically segregate the virus seed train from the cell culture seed train. The virus seed train can be done in single-use systems. The only limit to utilization of single-use systems would be for incubation of cell culture volumes above the typical single-use bioreactor sizes (2000L) and the associated harvest centrifugation of those large volumes.
BSL-3
Biosafety level 3 continues to build on BSL-2 and drives toward an entirely closed process. All manipulations must happen in a biological safety cabinet (BSC) or other physical containment. The facility needs HEPA filtration on vacuum lines, a method of decontaminating all waste leaving the area, a handwashing sink, and an eyewash station. A protective layer of clothing is to be donned before entering and removed on leaving the BSL-3 area. An airlock is required with airflow into the suite. There must be a way for personnel to verify airflow direction at the area entrance. The air can be recirculated, but only to the BSL-3 area, and all exhaust from the space must be HEPA filtered. Equipment that may produce infectious aerosols must be contained in primary barrier devices that exhaust air through HEPA filtration or other equivalent technology before being discharged into the laboratory area. Air exhausts must be situated away from air intakes. HEPAs need gas-tight isolation with decontamination ports and/or bag in/bag out capacity. Facility design should account for decontamination of large equipment that may have to leave the space. Showers for personnel leaving the space are an optional facility feature in the Biosafety in Microbiological and Biomedical Laboratories (BMBL), but may be required by other authorities. The BSL-3 space should be recertified annually.
There are few BSL-3 processes, and those typically have dedicated single-product facilities. The increased containment adds significant cost and drives the facility layout. The requirement to decontaminate all materials leaving the area results in large decontamination autoclaves at the BSL boundary. Frequently, the suites are designed to be isolated and fumigated. The complexity of dealing with showers and a handwashing sink in/adjacent to a classified space is a challenge brought about by applying laboratory standards to GMP facilities, but the risk of environmental monitoring counts can be reduced by using trap primers that feed disinfectant.
In general, the industry has been reluctant to embrace broad spectrum adoption of single use for BSL-3. One exception has been depth filtration. A single-use filter system can be pushed into an autoclave for decontamination, whereas steaming traditional stainless-steel housings is a challenge due to the bubble point pressure of the loaded filters.
An example of a BSL-3 process is development of a SARS coronavirus vaccine from the wild type virus, from viral bank through attenuation. The virus seed train could start in single-use systems, but would transition to stainless-steel systems once the volume exceeds what one person can easily handle (~20L 2D bag). Seals that contact the virus are typically flushed with clean steam or hot water for injection (WFI). Disc stack centrifuges are the hermetically sealed type or contain additional controls to mitigate aerosols. The bio-waste system is designed to be decontaminated by steam. Additional care should be taken in selecting instruments and electrical enclosures as the additional routine disinfections can take a toll on these installations and hamper any preventative maintenance activity.
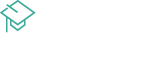
Are you expanding or renovating your facility to support Biocontainment efforts? Learn the basic principles of facility layout that allow your operations to scale without pain by attending Herman Bozenhardt and Erich Bozenhardt's webinar::
Improving Biological Facility Design: Critical Tips for Compliance
BSL-4
Biosafety level 4 processes are completely isolated inside primary barriers and extensive secondary barriers. These facilities are for basic research and are not used for commercial GMP production.
An example of a BSL-4 process is animal testing for efficacy of an Ebola vaccine candidate.
Risk Remediation And Control — The Layered Approach
In the previous sections, we discussed the various methods to contain biologicals in production facilities. Conceptually, you can think of each level of the BSL scale as denoting the number of containment envelopes needed. So, for BSL-1, we need one envelope; for BSL-2, we need two envelopes; and so on. At BSL-4, we should be providing three layers of containment, plus the personnel should either be completely enclosed in a specialized suit with its own breathing apparatus or manipulations must be done remotely by a robot.
As a practical matter “envelopes” are achieved through architectural/HVAC means or equipment usage. These envelopes are complete containment mechanisms, such as:
- A biosafety cabinet for small volumes and small equipment
- A closed single-use disposable system
- A closed isolator system (flexible or rigid) with negatively pressured HVAC (HVAC in for sterility and HVAC out for exhaust isolation)
- A closed stainless-steel vessel system with an automated decontamination/deactivation and waste discharge system
- A room with walls, floors, and ceilings that can be cleaned and decontaminated, HEPA air supply (at the required GMP grade), HEPA air exhaust, and having air locks on all entrances and exits. The envelope is both architectural and HVAC in this case; one cannot exist without the other functioning properly. The HVAC maintains the integrity of the space.
- Additional rooms can be added to provide additional envelopes only if they are contained inside of each other, not in series, as the normal flow of the process.
These envelopes become the building blocks of how to develop a process containment model within the traditional GMP design strategy. GMP design functions also must accommodate the envelope concept and retain the EU directives, as well as some additional features:
- Unidirectional flow of all process elements such as personnel, raw materials, intermediates, products, equipment, and waste must be maintained. In the case of biological containment, this unidirectional flow must include: 1) a decon autoclave for waste out, and 2) an internal decon station for equipment and personnel.
- An HVAC system at BSL-2 and above must be HEPA in/HEPA out. The BSL-2 air can be recirculated to the BSL-2 area if it is HEPA filtered.
- At BSL-2 and above, redundant backup air handling units (AHUs) or independent exhaust fans are required, in case one AHU fails; the differential pressure maintains the integrity of the envelope.
- At BSL-2 and above, the production facility will require uninterruptable backup power to provide power for the production envelopes. These will include the HVAC systems, process control instruments and systems, personnel safety systems, BSCs, and other designated critical processing equipment.
- Traditional air locks are used; however, there is a critical twist in the scheme. Modern biotech facilities use the “air bubble” scheme for the air locks. In this instance, the air lock is a positive pressure to both the lower EU grade and the higher one. For example, a grade D corridor would have a lower pressure than the air lock, and the air lock would also be at a higher pressure than the EU grade C room one might be traveling into. This scheme isolates each room and prevents contamination from the grade C room from entering a common corridor. In the case of BSL products, the air locks are made as an “air sink,” where the air lock is negative to the grade D space and the adjacent grade C space. This prevents any contamination from leaking out, regardless of how the doors are manipulated. This is also in keeping with the CDC guidelines requiring a negative cascade.
- The negative cascade must be designed at all EU classifications out to the controlled non-classified (CNC) area. This is to assure that in any catastrophic circumstance, the biological material, its particles, etc., move inward to the facility and not into the public or the environment. The negative cascade inward will lead to issues meeting classifications unless extensive sealing is designed and built in.
- Last, to add one level of complication, the EU and FDA have been asking a seemingly harmless question when faced with an air sink, especially between a grade D corridor and a grade C room. The question asks, “What is the grade of the air sink/air lock room?” If you answer grade D because the grade D corridor air enters it, then you have no air lock from the grade D area to the grade C processing area. If you answer grade C, you have the same paradox as before. The solution is to add one additional air lock between the air sink (for the CDC/NIH) and the grade C space to satisfy the FDA and EU. In this case the air sink is a true grade D separation zone, and the next air lock cascades air from the grade C processing room.
In summary, the normal GMP and EU design guidelines (see ISPE design guides) are followed; however, the NIH guidelines provide enhancements to the design. The key design features the NIH focuses on are those that will maintain the containment equipment, systems, and facilities under all conditions, especially failure. In addition, personnel safety and exposure are designed to be fault tolerant.
References:
- Vince McLeod, “Biosafety Levels 1, 2, 3, and 4”, Lab Manager, December 2010.
- The National Institutes of Health, Office of Research Facilities, Division of Technical Resources, Design Requirements Manual, revised December 18, 2012.
- Centers for Disease Control and Prevention, Biosafety in Microbiology and Biomedical Laboratories (BMBL) 5th Edition, December 2009.
About The Authors:
Herman Bozenhardt has 41 years of experience in pharmaceutical, biotechnology, and medical device manufacturing, engineering, and compliance. He is a recognized expert in the area of aseptic filling facilities and systems and has extensive experience in the manufacture of therapeutic biologicals and vaccines. His current consulting work focuses on the areas of aseptic systems, biological manufacturing, and automation/computer systems. He has a B.S. in chemical engineering and an M.S. in system engineering, both from the Polytechnic Institute of Brooklyn.
Erich Bozenhardt, PE, is the process manager for IPS-Integrated Project Services’ process group in Raleigh, NC. He has 11 years of experience in the biotechnology and aseptic processing business and has led several biological manufacturing projects, including cell therapies, mammalian cell culture, and novel delivery systems. He has a B.S. in chemical engineering and an MBA, both from the University of Delaware.