A Short Guide On Transitioning From Monoclonal Antibody To AAV Separation Science
By Adam L. Washburn, director of research and development, Eli Lilly and Company
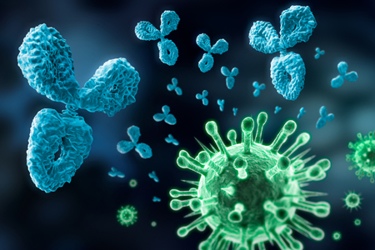
Adeno-associated virus (AAV) gene therapy candidates are becoming increasingly common in the biopharmaceutical clinical trial world. Although there are only a few approved AAV gene therapy products on the market today, the number of candidates in clinical trials is increasing. A search on clincialtrials.gov in January 2023 showed ~200 clinical trials associated with the term "adeno associated virus," with about half of those trials starting sometime after 2018.1 These numbers, however, are a fraction compared to other biotherapeutic clinical trials. For example, searching with the term "monoclonal antibody" results in a list of ~7,000 studies.
For companies interested in creating AAV gene therapy treatments, a large portion of the biopharma talent pool is much more likely to have prior experience with monoclonal antibody treatments than with AAV gene therapy treatments. As a result, many of the scientists engaged in creating AAV gene therapy products will likely be crossing over from a monoclonal antibody background, including many scientists involved in the development of analytical separations methods.
Fortunately, this crossover provides some advantages in understanding and interpreting analytical separations data for AAV therapeutics. Scientists who have transitioned from monoclonal antibody drug development to AAV therapeutics will be very familiar with techniques such as size exclusion chromatography (SEC), capillary gel electrophoresis (CGE, also referred to as CE-SDS), and ion exchange chromatography (IEX). Experience with these techniques allows prior knowledge to be leveraged from the monoclonal antibody realm into the AAV arena.
However, this familiarity can also present some roadblocks. Not all analytical knowledge can be directly transferred between these two different therapeutic modalities. In fact, there are some notable nuances that can make monoclonal antibody analytical experience a potential roadblock to correctly interpreting AAV analytical data.
This article discusses a few of those potential roadblocks and lays out some suggestions for appropriately transferring knowledge between therapeutic areas. SEC, CGE, and IEX assays will be discussed, and the similarities and differences between antibodies and AAVs in these assays will be highlighted.
Size Exclusion Chromatography
SEC is the gold-standard quality control separation method for measuring protein aggregates. It has been used for years to measure soluble aggregates of monoclonal antibodies.2 Through years of experience, complementary techniques such as analytical ultracentrifugation3 and light scattering4 have helped verify the results of size exclusion chromatography for protein therapeutics in determining levels of aggregates in monoclonal antibodies, as well as some fragment species.
Since measuring aggregates for monoclonal antibodies by SEC is well understood, it may seem like a simple task to transfer the technique from antibody to AAV therapeutics. In some respects, the transition is straightforward. With the larger hydrodynamic radius, AAV SEC separations simply require a chromatography resin with larger diameter pores. Once this factor is adjusted, the same mechanism and rules of separation can be employed as for antibodies.
However, there are a few subtleties with AAV SEC separations that can prove challenging or problematic compared to antibody separations.
One challenge that can arise is identifying species eluting prior to the main peak. In standard antibody SEC separations, this area is typically considered "aggregate space." However, for AAVs, soluble aggregates and free DNA impurities show up in this region. Even though the genomic DNA for an AAV is typically lower in molecular weight than the AAV capsid, its linear configuration gives it a higher hydrodynamic radius. This results in DNA eluting earlier than the spherical AAV particle. Consequently, the "aggregates" seen in an AAV SEC chromatogram can often contain free DNA in addition to viral capsid aggregates. Thus, peak identity cannot be taken for granted based on elution order.
Another challenge occurs with species eluting after the main peak. AAVs can easily dissociate from 60-mer capsids into capsid protein monomers. In principle, this large size difference should make it easy to separate and identify capsid monomer species from the fully assembled AAV capsid. In practice, however, there are some difficulties with this.
First, because the pore size of the SEC resin will usually be optimized for separating aggregates, the separation between intact capsids and smaller species will often be compromised. In many cases, a separation optimized for aggregates will result in monomer species co-eluting with species in the total inclusion volume or “buffer peak” of the SEC chromatogram.
Second, for some AAV serotypes, the AAV monomer proteins can tend to behave as “sticky” proteins. This can manifest in low recovery of monomer capsid proteins or in the elution of capsid proteins after the total inclusion volume due to protein-column interactions. As with the aggregate species, in this scenario the elution time does not fully align with the molecular weight or even the hydrodynamic radius of the species.
Fortunately, SEC separation and detection have several enhancements that can be added to help with these more complicated separations. DNA-specific peaks can typically be discerned by multi-wavelength absorbance ratios (e.g., A260/A280) or by looking for protein-specific fluorescence.5 As a result, SEC can potentially provide information about aggregation, free DNA, and empty/full ratios with just one run. Coupling with a MALS detector can allow for even greater characterization information.6 However, the nuances of each measurement moiety need to be well understood to avoid misclassifying or misreading the chromatographic data.
Capillary Gel Electrophoresis
A classic technique for measuring antibody protein purity is the SDS-PAGE gel. The simplicity of the instrumentation setup makes this a go-to technique for protein fragment analysis in both academic and industrial labs. AAV analysis has been no exception to this.
Despite the ease of setting up and running a gel, gel-based analysis can suffer from inconsistencies in elution, staining, and quantitation. As a result, modern control strategies for monoclonal antibodies have largely replaced SDS-PAGE gel analysis with capillary gel electrophoresis (CGE or CE-SDS). CGE provides more consistent separations that are appropriate for a quality control setting for antibody fragment analysis.7 Similarly, AAVs can be analyzed by CGE instead of by SDS-PAGE.8
For antibodies, CGE can be run in two modes: reduced and non-reduced. Reduced CGE breaks all disulfide bonds and allows for analysis of heavy and light chains as well as any fragments that form. Variants such as non-glycosylated heavy chain can also be separated. Antibody analysis by reduced CGE also provides information about heavy versus light chain ratios. However, with modern antibody production processes, heavy/light chain ratios typically remain at a consistent 1:1 ratio.
Non-reduced CGE for antibodies is helpful for measuring any covalent aggregation and any fragments that would not be discernible post-reduction such as the presence of half-antibody fragments.
For AAV therapeutics, CGE can also be used to analyze fragment formation. However, unlike mAbs, there are typically no disulfide bonds holding the AAV capsid superstructure together. As a result, there is little difference in reduced versus non-reduced CGE. In general, the denaturing conditions of either mode of CGE break the AAV apart into the three constituent monomer components (VP1, VP2, VP3) and any fragments that might be present.
Like antibodies, CGE analysis of AAVs provides two sets of information. First, fragment analysis can be determined by CGE. Fragments of the AAVs will typically elute earlier in the profile than intact monomer proteins. Truncated forms or variants of each of the monomer capsids show up as impurity peaks alongside the major VP1, VP2, and VP3 peaks. Because the VP peaks all elute closely together, separating these closely related variants can be somewhat more challenging than what is typically seen for monoclonal antibodies.
Second, CGE can also be used for monitoring the ratio of the monomer capsids VP1, VP2, and VP3. Unlike antibodies, AAV capsids do not form in strict stoichiometric ratios. Instead, these ratios can vary based on cellular processes that can vary depending on serotype, cell type used, growth conditions, and other factors. Because the capsid ratio can potentially impact viral infectivity efficiency, CGE analysis for capsid ratio can be an important quantity to measure, understand, and control.
Overall, CGE is an important analytical technique for both antibodies and AAVs. Knowledge of the technique for one modality can be extremely helpful in the execution and interpretation of the assay for the other modality. However, understanding how AAV CGE analysis differs from antibody analysis is a key to successfully executing CGE analysis for AAVs.
Ion Exchange Chromatography
Ion exchange (IEX) chromatography is a standard technique for assessing charge heterogeneity of monoclonal antibodies. Either cation or anion exchange chromatography can be used depending on the isoelectric point of the molecule. This technique helps identify protein modifications that impact the surface charge of the antibody, such as deamidation, isomerization, oxidation, or fragmentation.9
IEX can identify impurities of concern for a monoclonal antibody therapeutic as well as the presence of variants that do not directly impact the potency or efficacy of the molecule. Monitoring by IEX helps ensure that the process is consistent and that no significant chemical changes are taking place that would impact the overall potency and efficacy of the molecule.
For AAV therapeutics, IEX can be used in a similar sense — to observe molecular heterogeneity. However, the specific aspects of this technique diverge from antibody use.
Anion Exchange Chromatography (AEX) is a common separation technique used for AAVs to separate empty capsids (little or no encapsidated DNA) from full capsids (complete encapsidated therapeutic DNA) in the purification process. A similar technique can be used in an analytical setting.10 It should be noted that the separation efficiency can vary greatly between capsid serotypes. AEX is also not efficient at separating partial capsids (encapsidated truncated or variant DNA impurities) from full capsids. However, compared to other techniques such as analytical ultracentrifugation, AEX can provide a “quick read” on the empty/full ratio of a sample with minimal sample required for analysis.
Like antibodies, IEX can also potentially provide some understanding of the change in protein charge state due to amino acid modifications of AAV monomer proteins. Because these modifications could potentially impact the infectivity of the viral capsid, these alterations are important to characterize. In the literature these variations have often been characterized by mass spectrometry methods. However, AEX or Cation Exchange Chromatography (CEX) show promise for characterizing modifications such deamidation or oxidation.
One of the nuances with IEX characterization of AAV capsids, however, is that a 60-mer capsid will respond in a more subtle way to individual modifications than what is seen with a dimer antibody. Often, a single amino acid modification on an antibody can become visible as a new peak in an IEX chromatogram. For a 60-mer AAV capsid, however, a change to one amino acid impacts a much smaller percentage of the amino acids on the surface of the protein superstructure. As a result, modifications on intact capsids tend to show minor shifts in the peak elution time rather than appearing as new peaks.
Once again, the similarities between antibodies and AAVs are balanced against the different behaviors they exhibit in the same technique.
The Future Of AAV Separation Science
This overview covered three individual techniques. These workhorse protein separation techniques used for antibodies will continue to be used for AAV characterization. Having a knowledge of the nuances of these techniques will be helpful for scientists transitioning from monoclonal antibody work to AAV therapeutic work.
But what does the future hold for AAV separations? Will future work simply mirror antibody separation work?
While this article focused on protein separations, it is notable that an important aspect of AAVs is their genomic moiety. AAV DNA separations are an area ripe for future discovery, discussion, and analysis. Future articles and publications in this area will be a welcome addition to the field.
It is also clear that simply using the techniques of the past, while helpful, will not be sufficient to fully understand the complexity of AAVs. Additional development of advanced separation techniques will be crucial for thorough AAV characterization. Electrophoresis techniques for AAVs are an exciting area of development, as are 2D-LC separations.11
In conclusion, for a scientist migrating from monoclonal antibody therapeutics to AAV therapeutics, the transfer of knowledge from one field to the other will be useful. However, moving forward, the most important transfer will be the innovation and scientific knowledge used to create the standard techniques for monoclonal antibodies in the first place. This same spirit of innovation and discovery will be crucial to further expanding understanding and analytical capabilities available for AAV therapeutics.
References:
- https://clinicaltrials.gov/, accessed Jan. 2023. Search terms placed in the "Other terms" search box.
- Ricker, R. D., Sandoval, L. A., Fast, reproducible size-exclusion chromatography of biological macromolecules. Journal of Chromatography A 1996, 743 (1), 43-50.
- Krayukhina, E., Uchiyama, S., Nojima, K., Okada, Y., Hamaguchi, I., Fukui, K., Aggregation analysis of pharmaceutical human immunoglobulin preparations using size-exclusion chromatography and analytical ultracentrifugation sedimentation velocity. Journal of Bioscience and Bioengineering, 2013, 115 (1), 104-110.
- Sahin, E., Roberts, C.J. (2012). Size-Exclusion Chromatography with Multi-angle Light Scattering for Elucidating Protein Aggregation Mechanisms. In: Voynov, V., Caravella, J. (eds) Therapeutic Proteins. Methods in Molecular Biology, vol 899. Humana Press, Totowa, NJ.
- Meng, H., Sorrentino, M., Woodcock, D., O'Riordan, C., Dhawan, V., Verhagen, M., and Davies, C. Size Exclusion Chromatography with Dual Wavelength Detection as a Sensitive and Accurate Method for Determining the Empty and Full Capsids of Recombinant Adeno-Associated Viral Vectors. Human Gene Therapy 2022, 33:3-4, 202-212
- McIntosh, N.L., Berguig, G.Y., Karim, O.A. et al. Comprehensive characterization and quantification of adeno associated vectors by size exclusion chromatography and multi angle light scattering. Sci Rep 2021, 11, 3012.
- Zhu, Z., Lu, J., Liu, S. Protein separation by capillary gel electrophoresis: A review. Analytica Chimica Acta, 2012, 709, 21-31.
- Oyama, H., Ishii, K., Maruno, T., Torisu, T., Uchiyama S. Characterization of Adeno-Associated Virus Capsid Proteins with Two Types of VP3-Related Components by Capillary Gel Electrophoresis and Mass Spectrometry. Hum Gene Ther. 2021, 32, 1403-1416.
- Khawli, L., Goswami, S., Hutchinson, R., Kwong, Z., Yang, J., Wang, X., Yao, Z., Sreedhara, A., Cano, T., Tesar, D., Nijem, I., Allison, D., Wong, P., Kao, Y., Quan, C., Joshi, A., Harris, R., Motchnik, P. Charge variants in IgG1: Isolation, characterization, in vitro binding properties and pharmacokinetics in rats. MAbs., 2010, 2(6), 613-624.
- Wang, C., Mulagapati, S., Chen, Z., Du, J., Zhao, X., Xi, G., Chen, L., Linke, T., Gao, C., Schmelzer, A., Liu, D. Developing an Anion Exchange Chromatography Assay for Determining Empty and Full Capsid Contents in AAV6.2. Mol Ther Methods Clin Dev., 2019 15, 257-263.
- Wu, Z., Wang, H., Tustian, A., Qiu, H., Li, N. Development of a Two-Dimensional Liquid Chromatography-Mass Spectrometry Platform for Simultaneous Multi-Attribute Characterization of Adeno-Associated Viruses. Analytical Chemistry, 2022 94 (7), 3219-3226.
About the Author:
Adam Washburn, Ph.D., is a director of research and development at Eli Lilly and Company. His group supports bioproduct analytical development for monoclonal antibodies, peptides, and novel therapeutic modalities. His recent work has included analytical method development for AAV gene therapy.