Developing A Smart Inhaler For Optimal Drug Delivery
By Melissa Rhodes, PhD, DABT, Aerami Therapeutics
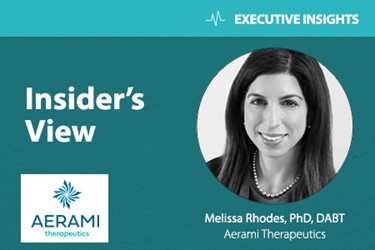
Historically, inhalation drug delivery was limited to the treatment and management of respiratory diseases, such as asthma or chronic obstructive pulmonary disease. However, this delivery approach has broadened as an alternative treatment option for a number of systemic diseases,[i] including endocrine and neurological diseases, which could benefit from the frequent pulsatile administration afforded by an inhaled delivery option.
Inhaled alternatives better mimic the physiological release of the desired compounds and allow for a faster absorption and onset of action compared to select oral or parenteral formulations. This could translate into better clinical outcomes and disease management.[ii],[iii],[iv],[v]
While the development and design of inhalers has improved significantly over the last decades, the use of inhaled therapies for treatment of systemic diseases has been limited by a number of factors, including complexity of device, inefficient drug delivery to the lungs, and side effects, which are attributed to the inhaler design and type of formulations used. These challenges impact adherence to treatment regimen, leading to low patient compliance and poor clinical outcomes.
Thus, there exists a need for more intuitive inhalation devices powered by technology able to aerosolize and deposit drug formulation deep into the lungs — improving treatment efficacy and patient experience.
Challenges associated with current inhalers and inhaled formulations
Limited lung deposition
The clinical effect of inhaled therapies is typically dependent on “lung dose,” the total fraction of the inhaled formulation deposited in the lung.[vi] This dose is determined by a number of pulmonary processes, which are in turn influenced by the type of device, aspects of the inhaled medication (such as drug formulation), and patient-related factors.[vii]
Lung dose is particularly relevant in the treatment of systemic disease, where the aim is to achieve a precise systemic drug concentration. A maximized drug deposition limits the amount of drug wasted. This is particularly important for manufacturers of the drug-device combo inhalers; if most of the drug makes it to the lung, less of it is manufactured, keeping costs down.
One of the main physiological barriers to successful delivery of drugs to the lungs is the impact of particles and droplets in the nose and mouth, which limits deep aerosol penetration to lung periphery. Patient behavioral characteristics, such as a poor inhalation technique, also can contribute to poor lung deposition.
For instance, passive inhalers, such as standard dry powder inhalers (DPIs), depend on the patient’s inhalation effort to achieve the correct flow rate that determines aerosolization of the powder and delivery of the desired dose. A number of DPI devices, together with a variety of lung formulations, have been developed to explore different challenges in lung deposition.[viii] This includes the development of Active DPIs that utilize an active aerosol engine with a low inhalation effort, which aim to achieve an increased lung deposition with reduced throat deposition. Still, most inhalers only deposit approximately 20-40 percent of the drug in the lung, meaning more than 60 percent of the drug does not reach the intended site.[ix]
A fundamental property that determines deposition of an inhaled drug in the lung or in the mouth-throat region is aerodynamic particle diameter.[x],[xi] Drug particles with aerodynamic diameters between 0.5 µm and 5 µm have the greatest chance to be deposited in the lung, with smaller particles within that range being deposited deep in the lung, such as the alveolar space.
Particles larger than 5 µm tend to deposit in the mouth-throat area.
Currently, nebulizer devices are typically able to deliver particles between 1 µm and 5 µm through the utilization of vibrating mesh technologies.[xii] However, there are a number of challenges associated with these devices. For instance, the drug delivery is typically completed in a continuous mode, independent of the patient’s inhalation/exhalation cycle. This leads to high dose variability and waste of drug into the air.
Similarly, pressurized meter dose inhalers (pMDIs) are able to aerosolize the drug to sizes of between 2 µm and 4 µm. However, these devices emit a fixed dose of medication with each pulse and eject the drug at high speed through the mouthpiece, leading to high deposition of the drug on the mouth/throat.[xiii]
Adverse effects
Another challenge with inhaled drug delivery is potential adverse effects related to device design or formulations. For instance, DPIs tend to form aggregates that are not respirable and therefore limit the amount of drug taken up via the lungs. These formulations usually contain a number of excipients to improve flow and aerosol dispersion of the powder. This is often associated with intolerability from patients (mostly cough) and may increase the risk of other adverse events, such as reduced lung function, over time.[xiv]
Increased inhaled therapeutic portfolio
As increasingly diverse inhaled therapeutics are explored, there is a need to develop devices that can deliver these new molecules while maintaining or improving efficacy and safety. New molecules being explored include a large variety of biologics, such as monoclonal antibodies or hormones with diverse molecule sizes, some of which have poor solubility and diverse aerosolization efficiency.
Many standard inhaler platforms — including DPIs, pMDIs, and nebulizers — face compatibility challenges and are not optimized for use with diverse molecules. Some of these devices will need to be redesigned to maximize delivery efficiency and efficacy while minimizing drug loss and side effects.[xv]
Optimizing Inhaled Drug Delivery
To increase lung dose and improve efficiency and patient treatment experience, the next generation of inhalers should meet the following requirements:
Technology that generates soft mist formulations
Aerodynamic particle diameter is key to achieving an optimal lung deposition. Some next-generation inhalers currently in development focus on the generation of soft and gentle mist formulations that enable deposition of the drug deep in the lung, reducing side effects related to particle aggregates and excipients, including cough.
An inhalation platform that uses vibrating mesh technology — with thousands of tiny, funnel-like holes that vibrate hundreds of thousand times per second — can push liquid formulations through the funnel, creating a fine mist of tiny droplets (3-6 µm). In addition to the inclusion of mesh technology, next-generation inhalers should be designed to allow for a laminar (rather than a turbulent) flow to achieve a total lung deposition of up to almost 80 percent[xvi] and fast onset of action. This enables precise and consistent delivery of the desired dose.
Compatibility with diverse therapeutic portfolio
Next-generation inhalers also must be compatible with a larger diversity of molecules than previous inhalers — including large molecules, such as fusion proteins (>200 KDaltons) or antibodies (>140 KDaltons).
Smart, intuitive devices that manage breathing flow and data
There is a trend toward designing Bluetooth-enabled smart devices that are more intuitive, driving patient engagement and adherence while increasing treatment effectiveness. As previously described, correct use of an inhaler is a key requirement for delivery of the prescribed dose — maximizing resources to reduce treatment cost. Next-generation inhalers in development incorporate digital interfaces to manage and adapt to patient’s breathing patterns. These devices understand the way the patient is breathing and coach him/her to breathe at the appropriate rate. This is particularly relevant in pediatric or geriatric patients.
Digital platforms and the internet are an integral part of people’s lives, so next-generation devices need to engage with connected devices and apps to provide patients with automated, real-time treatment data. This includes cloud-based database management, data analytic software, and personalized coaching tools to empower individuals with information to monitor their disease and make healthy behavioral changes.
About the Author
Melissa Rhodes, PhD, DABT, is Chief Development Officer for Aerami Therapeutics.
Melissa Rhodes has more than 15 years of drug development and biotechnology industry experience including almost a decade at GlaxoSmithKline. At Aerami, she oversees the prioritization and transition of key pipeline programs into human clinical trials. Prior to Aerami, Dr. Rhodes served as chief development officer at Altavant Sciences, where she oversaw the development of clinical assets, translational medicine, manufacturing and regulatory affairs. Before that, she held several safety assessment and non-clinical research positions at Roivant Sciences, GlaxoSmithKline and Erimos Pharmaceuticals.
Dr. Rhodes holds a Ph.D. in pharmacology and toxicology from Duke University Medical Center and is a Diplomate of the American Board of Toxicology. She can be reached at mrhodes@aerami.com
[i] Hickey, AJ. Back to the future: inhaled drug products. J Pharm Sci. 2013. 102(4):1165-72. doi: 10.1002/jps.23465.
[ii] Dang M, Koh AJ, Danciu T, Mccauley LK, Ma PX. Preprogrammed Long-Term Systemic Pulsatile Delivery of Parathyroid Hormone to Strengthen Bone. Advanced Healthcare Materials. 2016.6 (3): 1600901. doi:10.1002/adhm.201600901.
[iii] Cutfield WS., Derraik JGB, Gunn AJ, Reid K, Delany T, Robinson E, Hofman PL. Non-Compliance with Growth Hormone Treatment in Children Is Common and Impairs Linear Growth. PLoS ONE. 2011. 6 (1). doi:10.1371/journal.pone.0016223.
[iv] Patel AB, Jimenez-Shahed J. Profile of inhaled levodopa and its potential in the treatment of Parkinson’s disease: evidence to date. 2018. doi: 10.2147/NDT.S147633.
[v] Riddle M. Basal glucose can be controlled, but the prandial problem persists – it’s the next target! Diabetes Care. 2017. 40:291-300
[vi] Le Souef P. The meaning of lung dose. Allergy. 1999; 54, Suppl 49: 93-6.
[vii] Borghardt JM, Kloft C, Sharma A. Can Respir J. 2018; 2018: 2732017.
[viii] Yeung S, Traini D, Lewis D, Young PM. Dosing challenges in respiratory therapies. International Journal of Pharmaceutics. 2018. 548 (1): 659-671
[ix] Clark AR. Understanding Penetration Index Measurements and Regional Lung Targeting. Journal of Aerosol Medicine and Pulmonary Drug Delivery. 2012. 25 (4): 179–187. doi:10.1089/jamp.2011.0899.
[x] Tsuda A., Henry F. S., Butler J. P. Particle transport and deposition: basic physics of particle kinetics. Comprehensive Physiology. 2013;3(4):1437–1471. doi: 10.1002/cphy.c100085.
[xi] Edsbacker S., Johansson C. J. Airway selectivity: an update of pharmacokinetic factors affecting local and systemic disposition of inhaled steroids. Basic and Clinical Pharmacology and Toxicology. 2006;98(6):523–536. doi: 10.1111/j.1742-7843.2006.pto_355.x.
[xii] Itoga NK, Kinoshita CK, Masutani SM, Yamamoto LG. Mechanical factors affecting nebulized albuterol aerosol particle sizes for asthma drug delivery. AM J Emerg Med. 2014. 32 (6): 569-72. doi: 10.1016/j.ajem.2014.02.015
[xiii] Fernandez Tena A, Casan Clara P. Deposition of inhaled particles in the lungs. Archivos de Bronconeumologia. 2012, 48 (7): 240-246.
[xiv] Lasagna-Reeves, Cristian A., Audra L. Clos, Terumi Midoro-Hiriuti, Randall M. Goldblum, George R. Jackson, and Rakez Kayed. "Inhaled Insulin Forms Toxic Pulmonary Amyloid Aggregates." The Journal of Clinical Endocrinology & Metabolism. 2010. 95 (9): 4477. doi:10.1210/jcem.95.9.9994.
[xv] ONdrugDelivery Issue No 92, November 19th, 2018 .PULMONARY & NASAL DELIVERY
[xvi] Fink JB, Molloy L, Patton JS, Galindo-Filho VC, de Melo Barcelar J, Alcoforado L, Brandao SCS, de Andrade AD. Good things in small packages: an innovative delivery approach for inhaled insulin. Pharm Res. 2017. 34 (12): 2568-2578. doi: 10.1007/s11095-017-2215-2